2 History of Aircraft & Aviation
Introduction
The roots of aviation and aeronautical engineering can be traced back many centuries. However, only in the last century has humankind turned the dream of flight into a practical reality. Today, most people think nothing about getting on a jet airliner and flying at nearly the speed of sound for thousands of miles, perhaps even halfway around the planet in one flight. Nevertheless, getting to this point has required many innovative engineering solutions to overcome the challenges of building human-carrying vehicles suitable for fast and efficient atmospheric flight.
A demand for safer and more efficient designs for both military and civil use drives the continued development of new airplanes. Aeronautical engineering advancements have enabled larger and faster aircraft to carry more passengers and cargo, or other payloads, over longer distances. Military requirements also continue to challenge state-of-the-art aerospace technologies, such as improving the capabilities of occupied (piloted) and unoccupied aircraft (e.g., drones), targeting, surveillance, offensive weapons delivery, and defensive systems.
Looking back at the evolution of flight, which occurred over several centuries, it becomes clear that the most significant advances in aviation can be correlated to critical technological advancements, perhaps even to what might be called leaps or “quantum jumps” in those technologies. These jumps include advances in understanding aerodynamics, stressed skin metallic airframe design, lightweight and powerful engines, especially gas turbine and turbofan engines, propellers and other propulsive technologies, stronger and lighter materials made of composites, and, more recently, the use of computers and software engineering. The preceding list is by no means an exclusive list of advancements, with improvements in navigation systems such as GPS and collision avoidance, digital avionics, fly-by-wire flight controls, pilot training, and more stringent certification standards being intrinsically tied to all of the continuing and potentially future advances in aeronautics and aviation.
Learning Objectives
- Appreciate more about the historical evolution of airplanes and other aircraft types.
- Identify the critical challenges of flight and subsequent technological advancements in the history of aeronautics.
- Consider where the continued advances in aeronautics and aviation might take humankind in another half-century.
Early Ideas of Flight
Some of the earliest ideas of human flight can be found in Greek mythology. Icarus and his father, Daedalus, attempted to escape from the labyrinth of Crete by means of attaching wings to their arms, mimicking those of birds, which were constructed from feathers, threads, and beeswax. However, Icarus flew too close to the sun; the wax melted his wings, and he drowned in the sea. Nevertheless, according to the legend, Daedalus continued to fly and escaped successfully.
The ideas of mechanical flying machines can also be traced back to the Renaissance visionary Leonardo da Vinci, whose many notebooks (referred to as a Codex) date to the 15th century. To read his text (in Latin), it must be held up to a mirror and read backward, but even then, his unique writing style makes his words difficult to understand. Among da Vinci’s meticulously drawn sketches are flying machines and aeronautical contraptions, such as ornithopters with mechanically driven flapping wings to imitate the flight of birds, as shown in the image below. In his notebooks, he also shows an aerial screw device resembling a helicopter. In addition, Leonardo da Vinci thought about engine concepts, realizing humans could never produce enough power to achieve flight. However, he does not seem to have ever integrated his ideas of engines with his flying machine concepts.
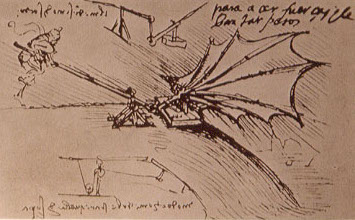
Toys in the form of kites, gas-filled balloons, and rotating-wing tops (known today as a bamboo dragonfly or bamboo-copter) were being used in ancient China around 2000 BC, with later attempts at building giant human-carrying kites. However, a hot-air balloon was the first recorded human-carrying vehicle capable of sustained flight. This lighter-than-air vehicle was designed in France by the Montgolfier brothers in 1783. Their first unoccupied balloon was made of paper and silk and rose to an altitude of about 2,000 m (6,000 ft). A few weeks later, a larger balloon named the Aerostat Reveillon was launched carrying pilot Jean-François Pilâtre De Rozier, who ascended to the end of its 90 m (250 ft) rope tether. A month later, De Rozier and the Marquis d’Arlandes made aviation history by conducting the first free flight in a balloon, which ascended to around 150 m (500 ft) and traveled approximately 8 km (5.5 miles) before safely landing.
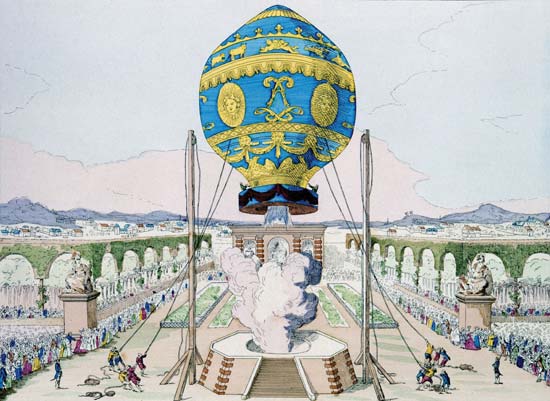
Benjamin Franklin witnessed some of these early balloon flights. He was so impressed that he predicted that balloons would soon have considerable military value for “conveying intelligence into, or out of, a besieged town, giving signals to distant places, or the like.” Indeed, tethered balloons were to be used during the American Civil War for battlefield surveillance. They could reach altitudes of up to 300 m (984 ft), giving a great vantage point for soldiers, who used signal flags to send information down to their commanders. Franklin later proposed lighter-than-air concepts combined with propulsion, eventually leading to the development of lighter-than-air aircraft or airships with rigid internal frames that became known as dirigibles. The word dirigible is usually associated with rigid-frame airships, the term originating from the French verb diriger (“to steer”).
The first dirigible was built and flown in 1852 by Henri Giffard and was driven by a propeller powered by a steam engine. Today, an airship is defined as any powered, steerable aircraft inflated with a lighter-than-air gas. Early airships and dirigibles were filled with hydrogen gas, which is highly reactive with many things, and airships had exploded even before the infamous Hindenburg disaster in 1937. A modern blimp, which is essentially a “pressure airship,” is a powered, steerable, lighter-than-air aircraft whose shape is maintained by the helium gas pressure inside the envelope. Unlike a dirigible, a blimp loses its shape when the internal gas pressure is lost. Newer versions of blimps now have a minimal internal structure made of carbon fiber, classifying them as semi-rigid airships.
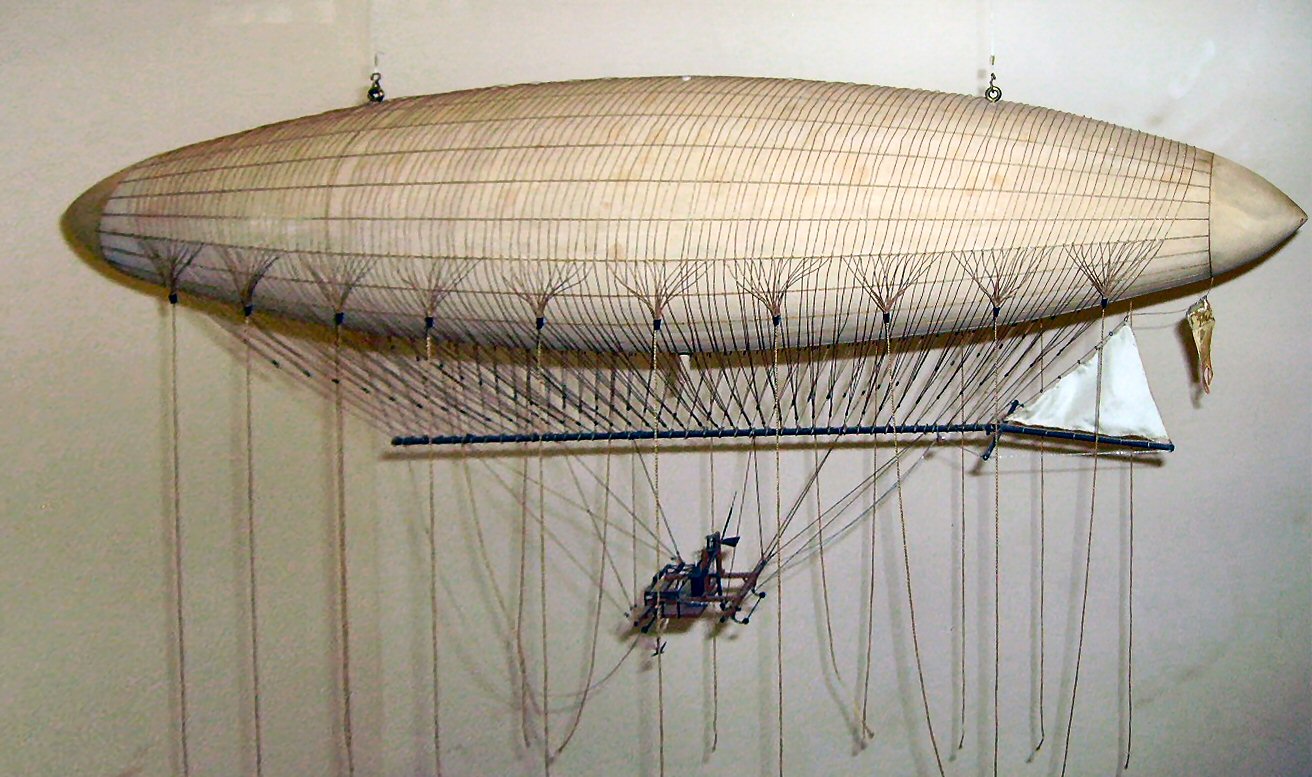
Invention of the Airplane
The sudden “invention” of the airplane is a misnomer because the origins of airplanes can be traced back several centuries. History suggests that the creation of a practical airplane was an evolutionary consequence of the contributions of many individuals over a relatively long period. Before the nineteenth century, there were few scientific investigations of flight or into the science of aerodynamics. Indeed, the earliest work on developing flying machines and airplanes can be placed into two distinct categories: inventive and scientific. The former is where intuition is used instead of formal technical training, whereas the latter is where a trained, more systematic approach is used. Before the twentieth century, nearly all developments in aviation were inventive, i.e., building something and trying to fly it, the usual outcome being failure. One does not need to understand a complex engineering system such as an airplane to make it work at its most basic level. However, understanding the underlying science is necessary to make it successful and practical.
Humankind has always been inspired by nature, especially in the form of birds. Even well into the twentieth century, there were attempts to build flying machines that emulated flapping wings. This thinking manifested in all sorts of weird contraptions, many in the form of ornithopters, an example being shown in the photograph below, all of which were unsuccessful. Nevertheless, many human-powered ornithopters, tower jumpers, and brave “bird-men” captured the public’s attention much more than any scientific endeavors at the time. Today, with the advantage of hindsight, most would agree that such efforts with ornithopters and flapping wing contraptions only served to derail the acceptance of more scientific thinking on what elements were needed to build a successful flying machine.
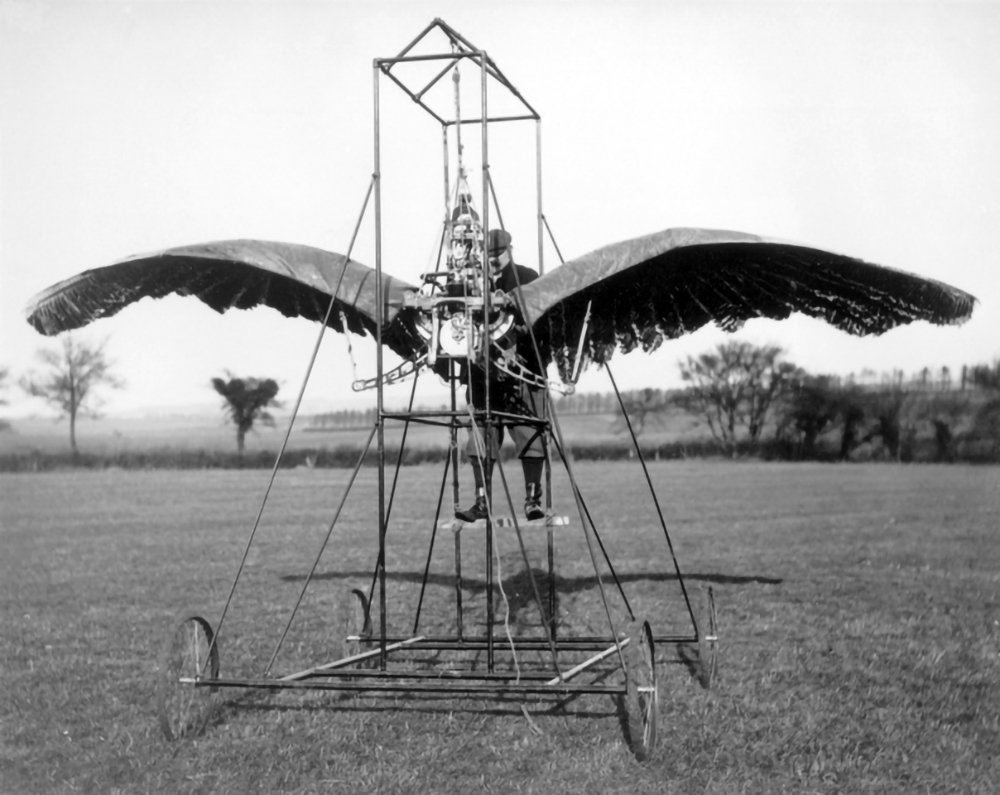
The inherent mechanical, aerodynamic, and other complexities in building a human-carrying aircraft with adequate power and control capabilities that did not structurally fail and fold up during flight resisted many efforts until the beginning of the twentieth century. Nevertheless, several fundamental issues needed to be overcome:
1. Understanding basic aerodynamics. The size of the wings and the power required to sustain flight were unknown to the earliest experimenters, who were guided more by intuition than science. While the science of aerodynamics, such as the force produced on a wing in a stream of air, had been explored by Isaac Newton and others, the first significant application of aerodynamic theory to airplane design occurred in the 1920s. The earliest airplanes did not have large enough wings to fly, or the wings were just too big and heavy, or they were so fragile they suffered structural failure.
2. The need for a suitable engine. This issue was a problem to be overcome at the beginning of the twentieth century by developing internal combustion (gasoline-powered) engines. Steam engines were never viable for any airplane, although attempts were still made to use them, often using a limited amount of superheated steam rather than carrying a boiler.
3. Designing a propeller. The ability to build a propeller suitable for aeronautical use was challenging. Early propeller shapes often emulated those used on oars or ships, which was not a good idea, even though the fundamental principles on which they work are the same. The best blade shapes for propellers that work efficiently in the air were determined through a long evolutionary process over many decades.
4. Obtaining structural strength and stiffness while minimizing weight. Minimizing the empty structural weight so the machine could take off and fly with a pilot and even a small payload was difficult, even with relatively lightweight wood and fabric. Early internal combustion engines were made of cast iron and were very heavy relative to their power output. Aluminum was not available commercially until about 1890 and was not used as a construction material in aeronautical applications until about 1915.
5. Providing stability and adequate control. Early aircraft were unstable or marginally stable, and many crashes occurred even though the machines may have been structurally sound. It took many decades to understand the critical connection between the center of aerodynamic lift on the machine and its center of gravity, as they both affected flight stability. Using effective control surfaces, especially for roll, took many years to develop.
At the beginning of the 19th century, George Cayley, an English nobleman and prolific scientist, proposed various aircraft concepts, including gliders, numerous types of airplanes, and even a primitive helicopter. His glider and airplane concepts used a primary wing for lift generation with smaller horizontal and vertical tail surfaces for directional stability and control. Cayley built many free-flying gliders at various scales, which he used to establish a basic understanding of aerodynamics and flight stability. He also coined the balance of four forces that influence aircraft flight, namely lift, weight, thrust, and drag, which later earned Cayley the title of the “Father of Aeronautics.”
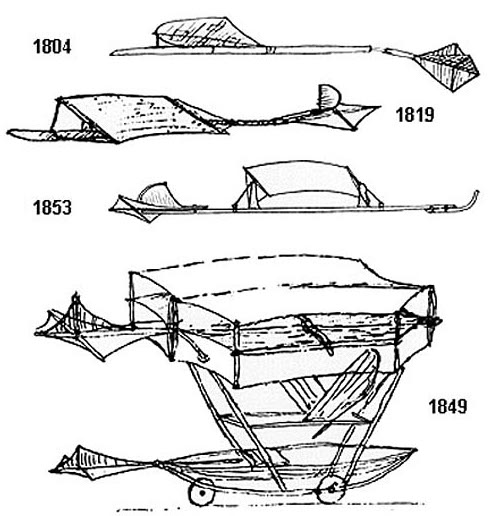
Cayley experimented with wing models placed on the end of a whirling arm device and correctly concluded that the aerodynamic forces produced on his models were proportional to the squared value of the speed at which the model moved through the air. Cayley also recognized the need for a lightweight engine to power his aircraft. However, in the mid-19th century, only steam engines were available, which were unsuitable for aeronautical use. Besides the heavy steam engine itself, it would have to carry along a boiler with ample water and fuel to make steam; this is impractical for an airplane because such an overall system would be enormously heavy.
In Germany, Otto Lilienthal developed several glider concepts, reportedly having conducted over a thousand flights from the top of a hill by the beginning of the 20th century. Octave Chanute, a Frenchman living in the U.S., furthered Lilienthal’s work. Chanute helped others, including the Wright brothers, with advice on the design of flying machines and also wrote a book on his experiences, which is still available today. Chanute’s open approach to discussing developments in the evolving aviation field ultimately led to considerable disharmony with the Wright brothers. The Wrights were secretive about their work and quickly patented their ideas to protect them, eventually getting into a significant “patent war” with others. However, it was to be the Wright brothers who were the first to achieve successful powered flight where others had previously failed.
First in Flight
In December 1903, the available historical record and the Smithsonian Institution documents that the Wright brothers, Orville and Wilbur Wright, were the first to fly a powered biplane aircraft that took off and landed under the control of its pilot, a photograph of the famous flight being shown below. Prior to 1903 the Wright brothers were experimenting with various biplane gliders, which laid the groundwork for their powered aircraft. There remain claims that Gustave Whitehead flew a powered aircraft several times between 1901 and 1902, pre-dating the first flights by the Wright brothers by over a year, but this is highly unlikely.
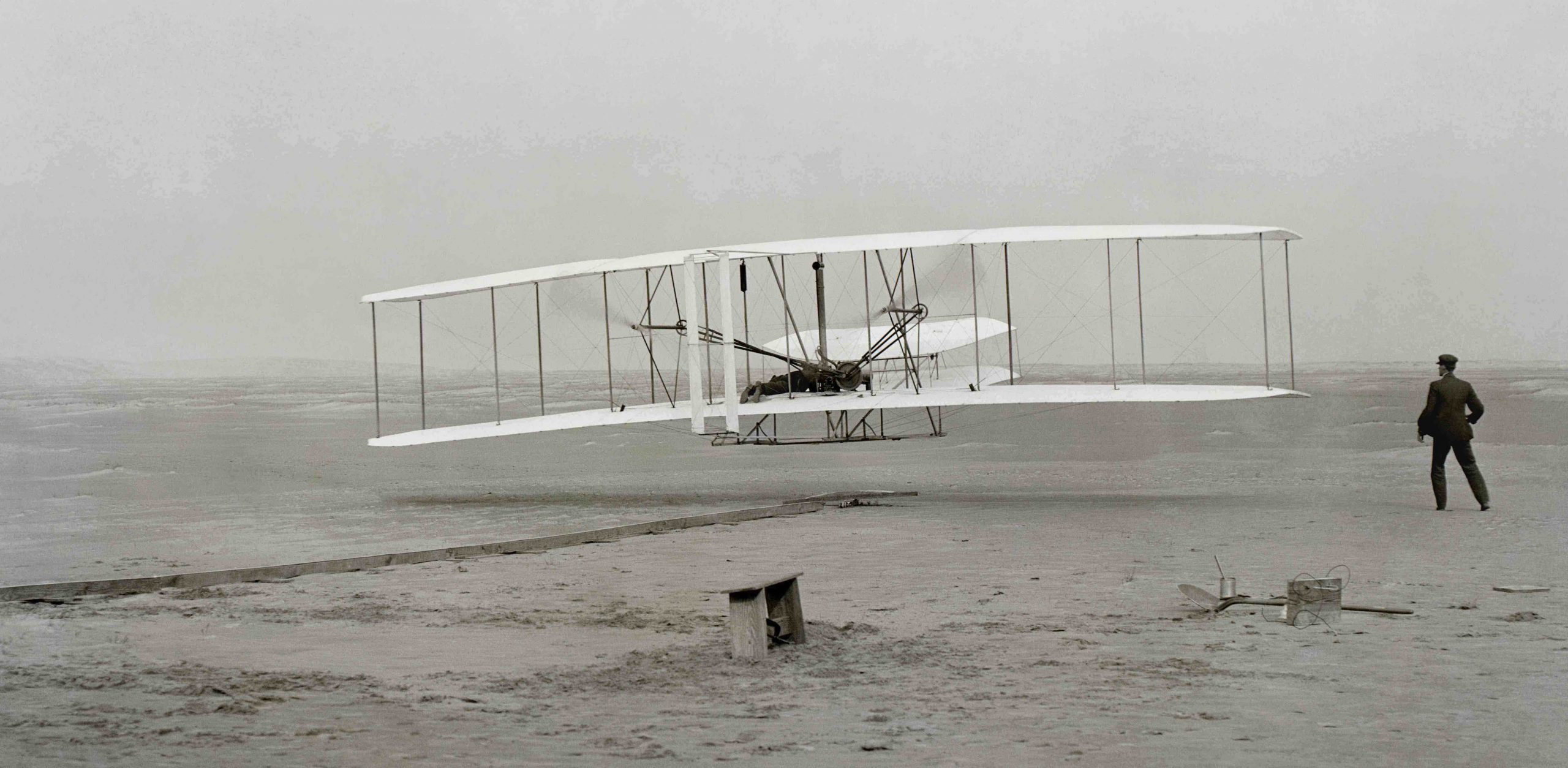
Shortly before this, Samuel Langley had tried to fly an aircraft with tandem (fore and aft) monoplanes, but the wings folded up in flight, and his machine crashed into the Potomac River. Aircraft structures must be strong and lightweight, but their limited stiffness means they can deform during flight under the action of the aerodynamic loads. Langley’s machine crashed because his monoplane’s wood and fabric wings were not stiff enough and broke off. The Wright brothers experimented with different types of structures and knew how to make a much more rigid structure in the form of a biplane.
Notably, the Wright brothers designed and built not only their airframe but also the engine and propellers to power their aircraft, i.e., they understood how to integrate the essential components of an airplane and then make it work as a system. They even built a wind tunnel, which they used to make systematic measurements of the aerodynamic forces on wings of different shapes, including the critical effect of aspect ratio, i.e., the beneficial effects of the slenderness of the wing on aerodynamic lift and drag. In this regard, they built their famous Flier with a wing aspect ratio that significantly exceeded anything else flown previously. Louis Breguet of France later made meticulous tests of wings airfoil shapes, paralleling those of the Wright brothers and reaching similar conclusions.
The Wright brothers continued to develop their aircraft designs for several years, and by 1908, their aircraft could fly for over two hours and cover nearly 100 miles. Wilbur died in 1912, but Orville lived until 1948. Orville witnessed many milestones in aviation, including the invention of the jet engine, but also saw the destruction caused by airplanes being used as weapons of war during WW1 and WW2. He said, “We dared to hope we had invented something that would bring lasting peace to the Earth. But we were wrong. We underestimated man’s capacity to hate and to corrupt good means for an evil end.”
Significantly in the history of aeronautics and aviation, the Wright brothers systematic engineering approach led to their continued success rather than the more inventive approach pursued by others trying to learn to fly and achieve powered flight. In Europe, Santos-Dumont later developed several powered aircraft based mainly on the Wright brothers’ successful concept, making the first public flight demonstrations of his aircraft in Paris in 1906.
Period 1910–1930
Several significant advances in aircraft development and aeronautical engineering occurred during and after WW1, and the developments in engine and propeller designs continued. The Wright brothers had shown that a propeller needed to have large aspect ratio blades with nose-down twist along the length of blade to achieve good propulsive efficiency, which was another key to their success. The “raked” propeller had more blade area toward the tip, as shown in the figure below, which gave further improvements in efficiency, allowing more of the engine power to be converted into useful thrust.
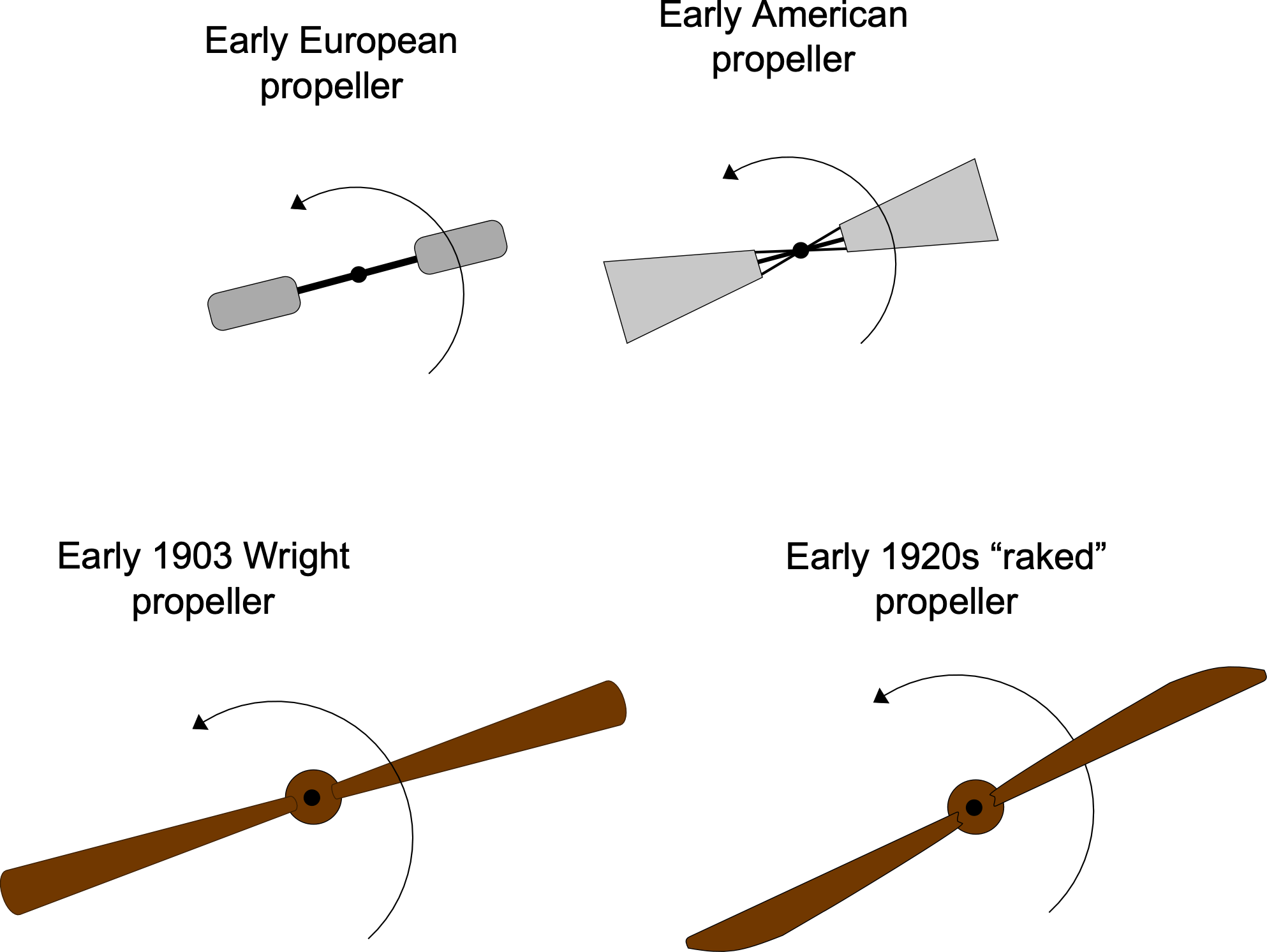
Although monoplane wing designs were slowly starting to appear, the biplane design saw various improvements, including methods of strengthening the wings and airframe and reducing the drag of the structural bracing and wires. Early airframes, however, were still made primarily of wood and covered with doped cotton fabric, wood being a readily available, durable, and easily worked construction material. However, such a construction method is limited because it becomes increasingly structurally inefficient and heavy for larger aircraft types.
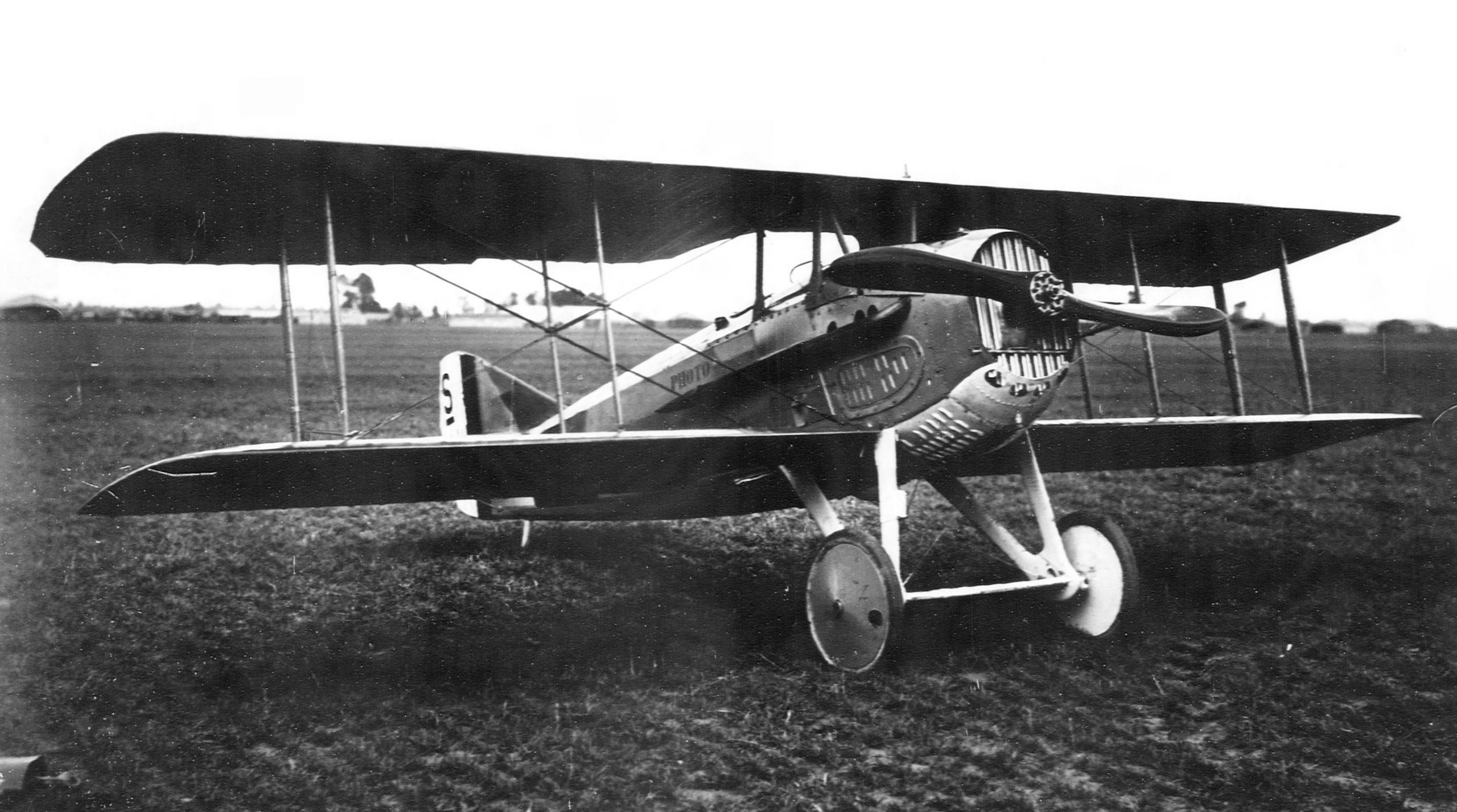
These early airplanes also used air-cooled rotary engines in which the crankshaft remained stationary, with the entire crankcase and its cylinders rotating with the propeller around the crankshaft. However, the rotary engine’s large rotating mass combined with the inertia of the propeller meant that it behaved like a giant gyroscope, causing various stability and control problems for the aircraft, e.g., the ability to turn only in one direction. These latter problems were mostly resolved using radial engines and other types of piston engines, such as “in-line” and “V” forms.
Increasing aerodynamic lift and reducing drag became prime objectives for aeronautical engineers to improve aircraft performance and maneuverability. By 1915, there was already significant interest in the monoplane wing, which offered airplane designers the potential of substantial reductions in drag and airframe weight compared to this era’s structurally braced biplanes and triplanes. For example, the Junkers J-1, as shown below, was not only an early monoplane but also pioneered metal airframe construction.
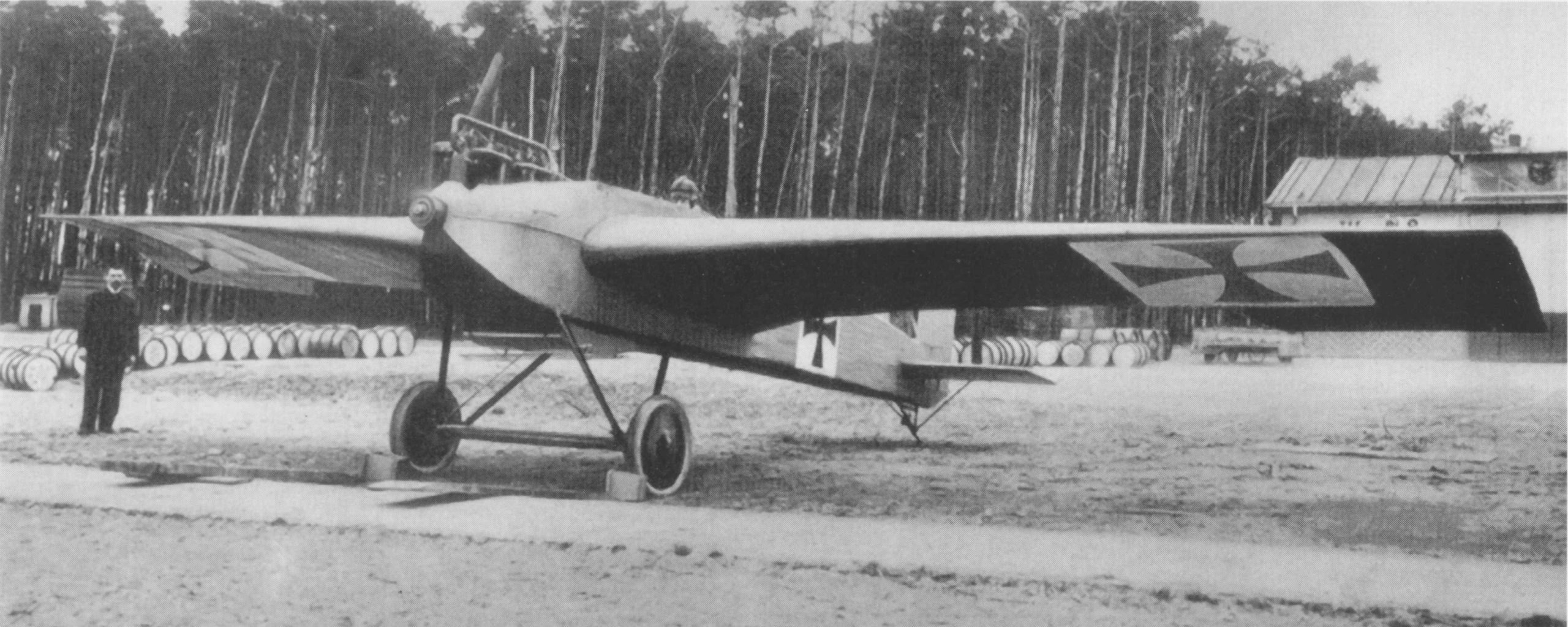
The earliest monoplanes were unsophisticated, with unrefined wing sections and rectangular wing planforms. In the quest for improvements, engineering interest peaked toward a better understanding of the aerodynamic effects of airfoil and wing planform shapes. This led to systematic wind tunnel investigations to measure lift and drag, some of which had been initiated by the Wright brothers using a small wind tunnel and force balance of their own design.
However, the continuing lack of rigidity of the early monoplanes led to the aeroelastic problem of wing flutter in high-speed dives and severe wing bending in even the most benign maneuvers. Flutter can result in catastrophic structural failure, and many accidents caused by wing and control surface flutter occurred during the 1920s and 1930s. These problems, however, were soon to be overcome with advances in understanding how to design stronger and stiffer aircraft structures, mainly by using aluminum instead of wood and fabric.
The end of WW1 saw aeronautical technology increasingly focused on civil air transportation goals, the first passenger-carrying aircraft being flying boats. In 1920, the American Curtiss NC-4 flying boat, which was powered by four 400 hp engines, became the first aircraft to cross the Atlantic, taking 16 days with many stops. Two weeks later, Royal Air Force pilots John Alcock and Arthur Brown made the first nonstop transatlantic flight in a Vickers Vimy, which took 15 hours and 57 minutes.
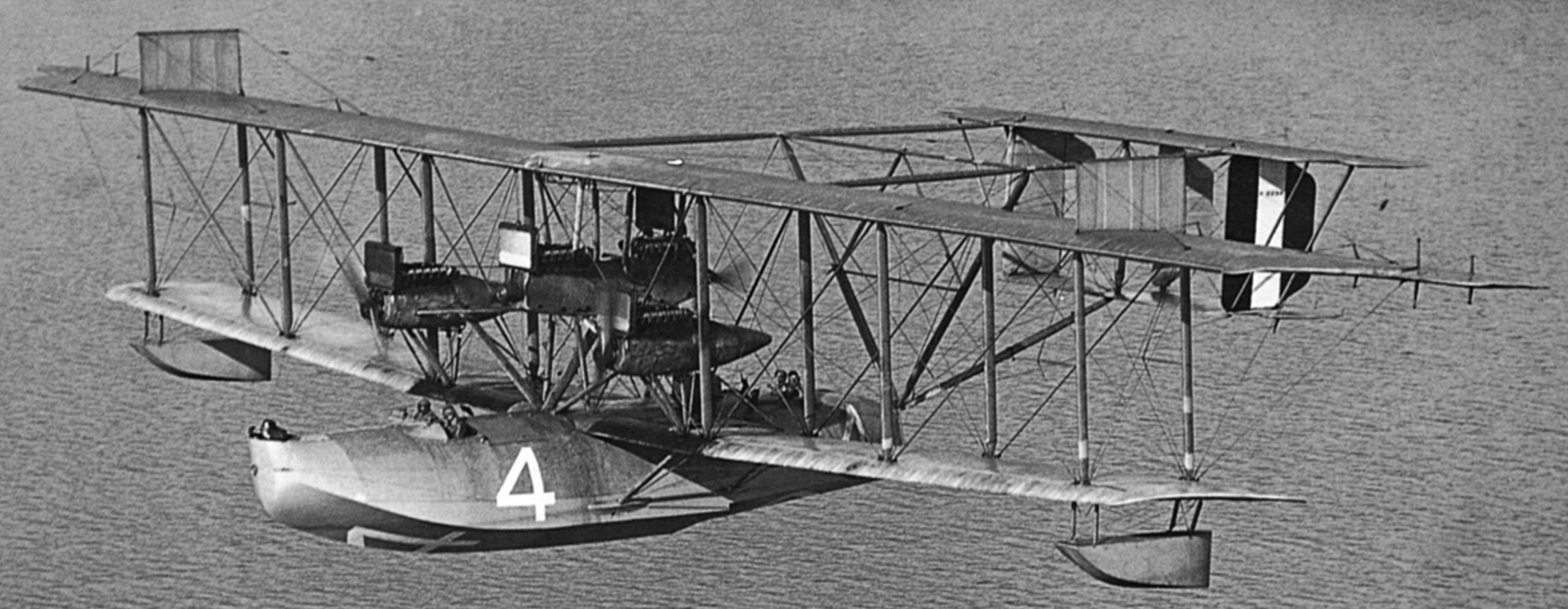
Charles Lindbergh made the first solo nonstop transatlantic flight from New York to Paris on May 20–21, 1927, in the single-engine Spirit of St. Louis. The British were soon to follow the Americans, flying various civilianized versions of bombers, including the Vimy. The use of flying boats for civil aviation, many of them being modified military aircraft, persisted into the 1950s, partly because of the lack of suitable airfields with refueling availability or those with long enough runways.
Period 1930–1950
There were many advances in aeronautical engineering leading up to and throughout WW2, including rapid engine technology developments. Concepts such as supercharging allowed for the development of high-powered piston engines. This led to dramatic improvements in overall aircraft performance, including much higher achievable flight speeds and flight at higher altitudes. By this time, most aircraft were of the more aerodynamic and structurally efficient monoplane type, the biplane all but being relegated to aeronautical history.
Further advances in metallurgy led to more powerful piston engines with improved power-to-weight ratios and better reliability. However, even then, early engines would run for only a few hundred flight hours, with valve and piston failures being common. Propeller technology also saw significant advancements in the 1920s, primarily in terms of propulsive efficiency. Using lightweight construction materials such as aluminum also began to be introduced into aircraft designs, allowing aeronautical engineers to develop stronger and stiffer airframe structures, lighter engines, and better propellers. Today, high-strength and lightweight aluminum alloys are vital to the success of the aerospace industry. However, aluminum was unavailable commercially in the alloyed form needed for aircraft construction until the late 1920s.
As power requirements increased, the rotary engine soon reached its design limits, not least because of the adverse gyroscopic effects it produced. Rapid advances in metallurgy and designs with better cylinder cooling finally allowed higher-powered radial engines to be developed. Fuels, too, saw better formulations to increase power output. Early types of gasoline were explosive and caused many engine failures because of pre-ignition. The use of lead tetraethyl (Tetraethyl lead) in the fuel (thereby raising the so-called octane number) allowed for a more controlled burn of the fuel (this is called “deflagration”), allowing higher compression ratios to be used, so more power could be obtained, and the engines were also more reliable.
The Wright 225hp (168kW) J-5 (R790) Whirlwind radial engine, as shown in the photograph below, was first produced in 1925 and is notable because it is considered one of the first reliable aircraft engines. Engines of that era would fail or need to be overhauled in less than 50 hours of operation. Nevertheless, the reliability of early aircraft piston engines remained relatively poor, and catastrophic in-flight failures were not uncommon.
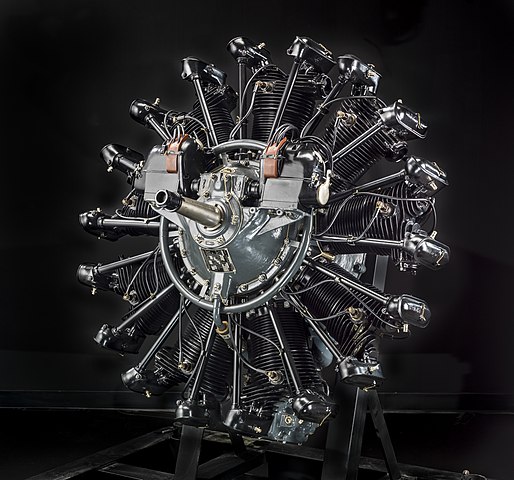
Propeller technology also saw more advancements during this period, including developing variable pitch or constant speed propellers. These propellers allowed their aerodynamic propulsive efficiency to be maintained over a wide range of airspeeds. Combining high-powered, supercharged reciprocating engines with constant-speed propellers and increasingly aerodynamically and structurally efficient airframe designs led to many successful military aircraft, including fighters and bombers.
By the 1930s, the development of lightweight airframe structures in the form of stressed-skin monocoque and semi-monocoque designs contributed to rapid improvements in aircraft range and payload-carrying capability. A monocoque design refers to a shell-like stressed skin mainly unsupported by any internal structure. A semi-monocoque design contains internal structures, including spars, ribs, stringers, etc., to prevent the thin skin from buckling, and this type is typical of most modern aircraft construction.
The all-metal Junkers Ju-52, a twin-engine German design, and the twin-engine powered American Boeing 247-D were to define a new era of civil air transportation that saw improvements in flight safety and passenger comfort. The distinctive corrugated skin of the Ju-52 gave the structure much strength and resistance to buckling with relatively low overall airframe weight. Other airplane designs were soon to follow as civil aviation expanded and airlines demanded larger airplanes from aircraft manufacturers that could carry more passengers.
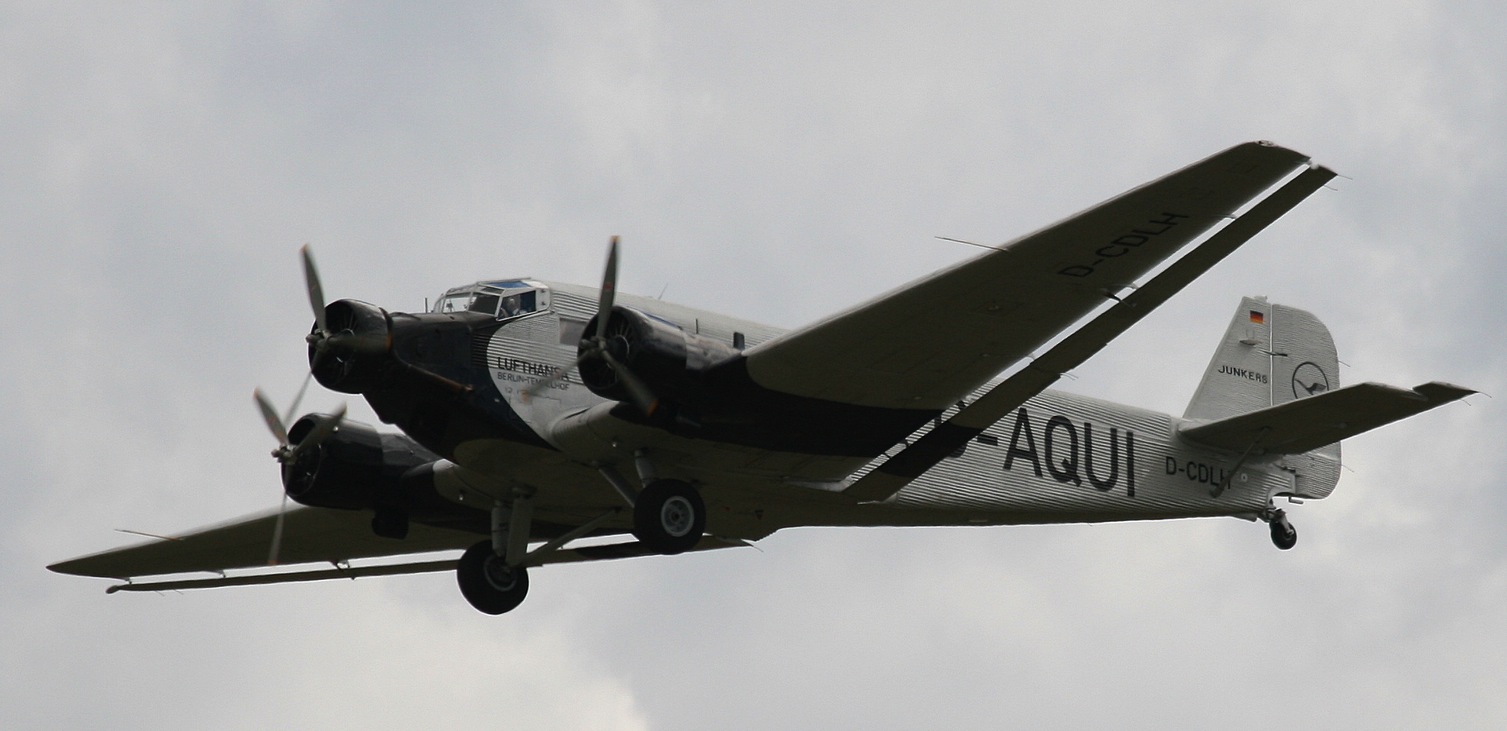
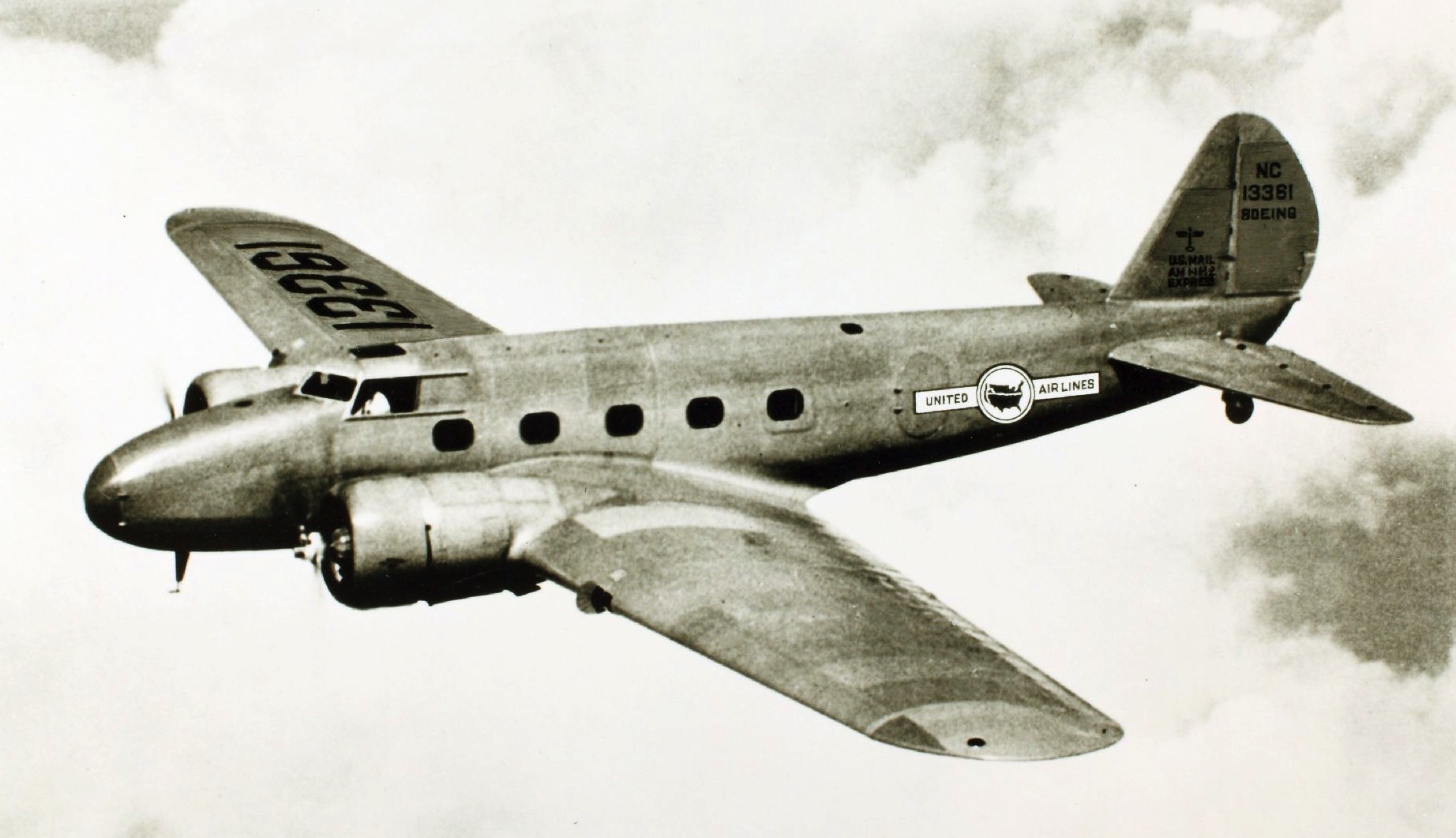
One of the 1930s and 1940s most famous aircraft was the Douglas DC-3, a twin-engined monoplane of all-metal, semi-monocoque stressed skin construction. It was a reliable and easy aircraft to maintain and was to provide a quantum leap in civil air transport capabilities. The DC-3 had a good flight range, even with a full load of up to 32 passengers, and could also operate from short runways and grass fields. The military version, the C-47 Dakota, saw much use during WW2 as a transport.
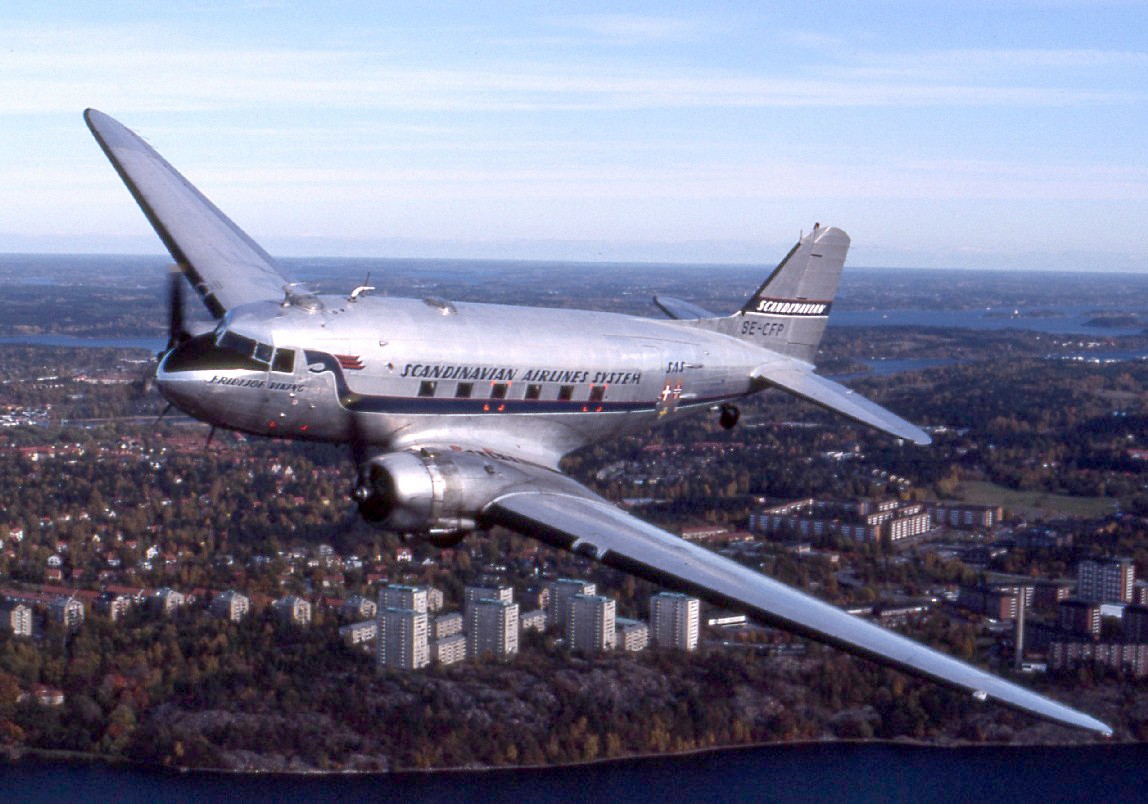
The British Supermarine Spitfire was one of the most technologically advanced fighters of WW2, combining advances in lightweight all-aluminum stress-skin airframe design with a powerful supercharged engine. The distinctive elliptical wing planform of the Spitfire had relatively low thickness and gave good aerodynamic efficiency and low drag. However, the early Spitfires suffered from low roll rates and aeroelastic problems such as roll reversal, which was associated with the low structural stiffness at the wing tip. While the shape of the wing meant that it took longer to build and was more expensive, the Spitfire was vital to the ultimate success of the Allies during WW2.
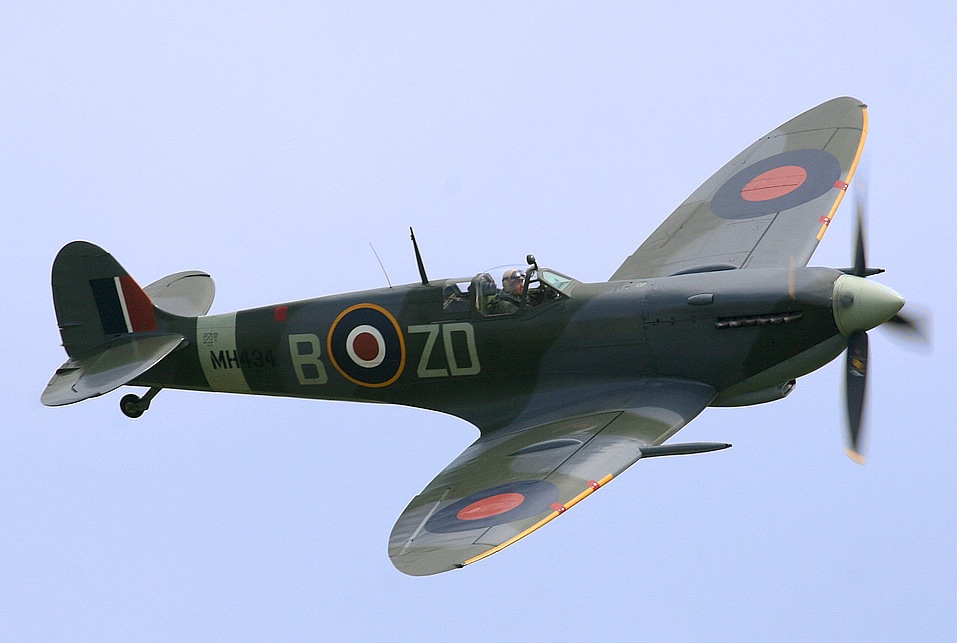
After WW2, many further strides were made in aeronautical engineering, which led to the development of increasingly larger and faster aircraft that benefitted both military and civil aviation. Civilianized transport aircraft were initially developed from flying boats and WW2 bombers, but it was only a short time before multi-engine aircraft were designed to carry passengers over considerable distances.
Larger transport aircraft were quickly developed by U.S. manufacturers, e.g., the Douglas DC-4 through DC-7 series. These were followed by even more capable transport aircraft, such as the Lockheed Constellation and Super Constellation with its distinctive triple tail, which became one of the most successful airliners of the 1950s. It was powered by four 18-cylinder, 3,250 hp piston engines and could carry up to 95 passengers in pressurized comfort above most of the weather at a cruising speed of about 300 knots. The Constellation was also used extensively by the military.
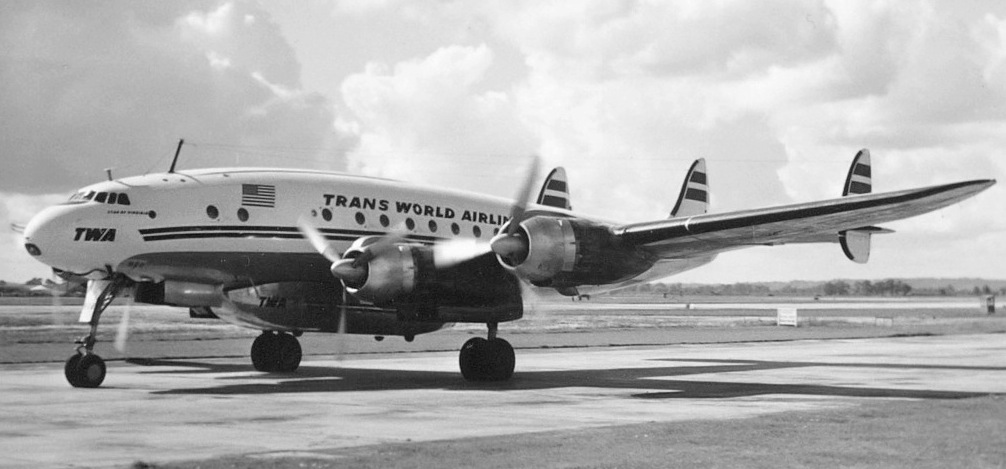
Development of Jet-Powered Aircraft
The first airplane to be powered by something other than a propeller was the Coanda-1910, designed by Henri Coanda. He referred to his propulsion system as a “turbo-propulsor.” But it was only a ducted fan with a centrifugal blower driven through a shaft by a piston engine, the air exhausting into a duct and so providing jet thrust. The aircraft reportedly took off, but it was underpowered and crashed.
Even in the early days of WW2, German and British aeronautical engineers were concurrently (but independently) working on the turbojet engine, which provided pure jet thrust. This engine type soon led to new aircraft designs with revolutionary flight capabilities. In 1929, Frank Whittle began work on his series of centrifugal gas turbine designs. Still, for various reasons, his first practical jet engine was not produced until 1937.
It was the German Heinkel 178 that was to make the first flight of a jet-powered aircraft, which was in late 1939. This jet engine, which burned diesel fuel, was designed by Hans von Ohain, who in 1935 had filed for a patent on the concept. However, Frank Whittle had formally patented the jet engine in 1930, seven years before Ohain’s patent application. For this reason, Frank Whittle is generally recognized as the “Father of the Turbojet Engine.”
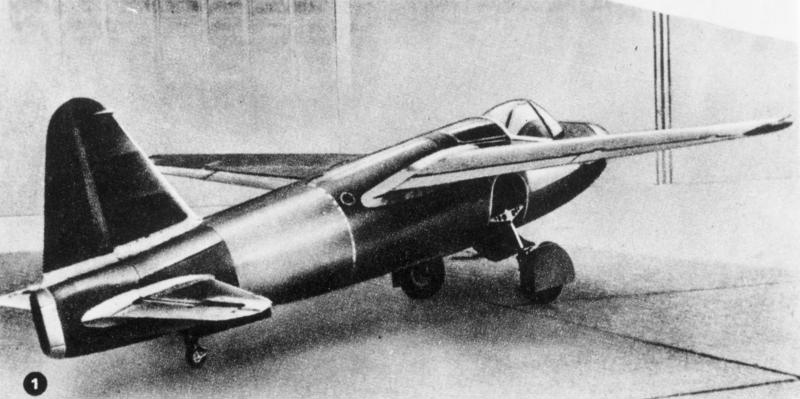
The first fully operational turbojet-powered aircraft was not introduced into service until 1944 in the form of the British Gloster Meteor and the German Messerschmitt Me 262 nicknamed Schwalbe (“Swallow”) in fighter versions, or Sturmvogel (“Storm Bird”) in fighter-bomber version. It was the Gloster Meteor, which was the first jet to down another aircraft. The first American turbojet-powered aircraft was the Bell P-59 Airacomet, which first flew in 1942. It was found to offer no operational advantage over existing propeller-driven airplanes and was never flown in combat. The next jet, the Lockheed P-80, reached operational flight status toward the end of 1945 and, like the Gloster Meteor, saw some limited use over Germany before the end of WW2. The P-80 was soon replaced by the highly successful swept-wing F-86 Sabre, for which nearly 10,000 aircraft were built.
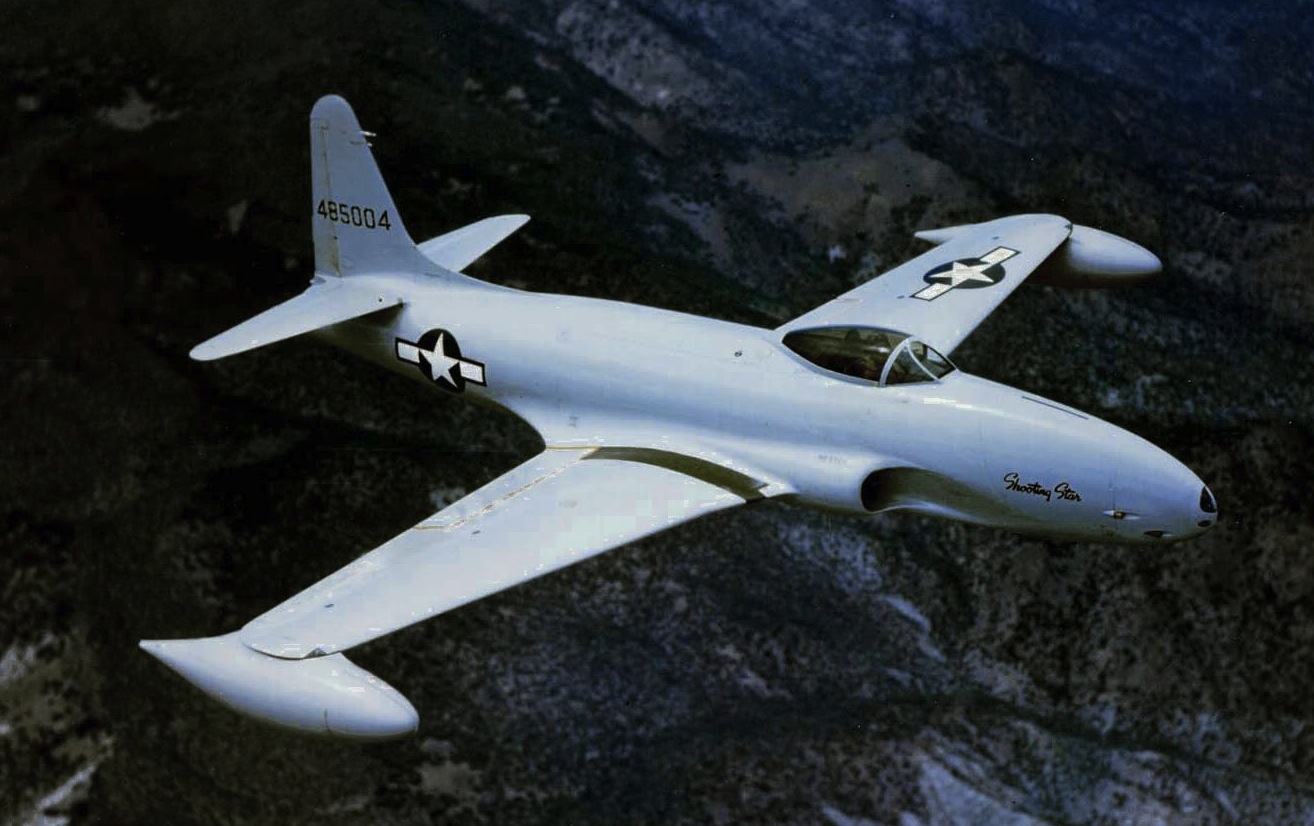
The invention of the turbojet engine soon led to the development of the axial flow turboshaft engine, designed to drive a propeller rather than produce pure jet thrust from the engine exhaust. The better performance, smooth running, quietness, and reliability of turboprop engines soon rendered most piston-engine powered propeller airliners obsolete. The increased performance and range of turboprop “jet” airplanes allowed for transcontinental and transoceanic flight in pressurized cabins in smoother air at higher flight altitudes. In 1953, the four-engined British Vickers Viscount would inaugurate the world’s first turboprop-powered airline service. The Viscount was sold to many airlines worldwide and remained operational until the mid-1970s.
In the U.S., the Lockheed Electra was a widely used medium-range 4-engine turboprop that saw airline service during the 1960s. However, the Electra model suffered two fatal crashes traced to a form of catastrophic wing flutter. The problem was identified as a pylon-whirl flutter instability, where a lack of structural stiffness in the engine mounts caused resonance with a torsional frequency of the wing. There is also a film of NASA flutter tests of an aeroelastically scaled model of the Electra in a wind tunnel. After the issues were fixed by stiffening the wings and engine mounts, the aircraft went on to be successful, but it was soon replaced on long-distance flights by newly developed jet aircraft such as the Boeing 707 and the Douglas DC-8. The military derivative, the P-3 Orion, was only recently retired in 2024 to be replaced by the Boeing P-8 Poseidon.
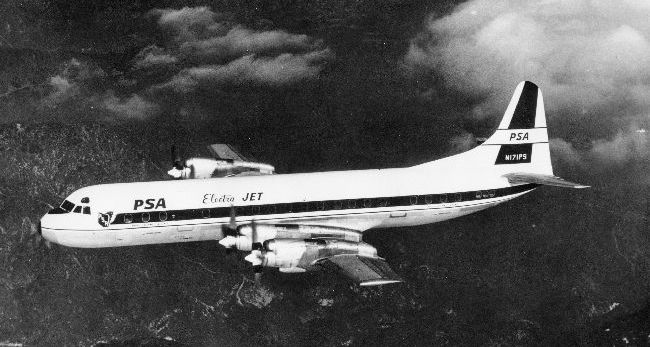
In early 1950, the turbojet-powered British de Havilland Comet inaugurated commercial jet aircraft transportation. This revolutionary aircraft was highly streamlined with a thin sweptback wing that contained four jet engines, allowing the airplane to cruise at subsonic Mach numbers of about 0.7. However, the aircraft soon suffered a series of sudden and catastrophic crashes and was withdrawn from service. Eventually, aeronautical engineers traced the cause of the crashes of the Comet to airframe failures and explosive decompression arising from metal fatigue originating at stress concentrations at improperly drilled rivet holes, especially around window cutouts; the original report explains the details.
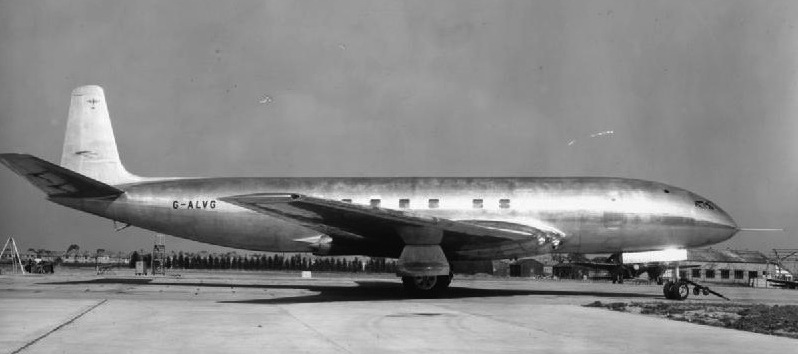
Metal fatigue is the weakening of a metal caused by repeatedly applied cyclic loads, typical of those found in flight structures, resulting in the formation of tiny micro-cracks and then visible cracks. The subsequent research and understanding of the metal fatigue problem led to significant improvements in the strength and durability of lightweight aluminum structures. For jetliners, rivet holes needed to be adequately prepared, which meant the elimination of “punch” drilling, as well as ensuring the deburring and secondary finishing of the holes before the rivets were installed. Despite the tragedy of the Comet crashes, which eventually resulted in another quantum leap forward in aviation technology. The redesigned Comet 4 saw airline service until the 1990s as well as military service with the Royal Air Force as the AWACS Nimrod.
By 1958, the Boeing 707 airliner was introduced into airline service and could make nonstop transcontinental and transatlantic flights. With its high aspect ratio swept wing, it could cruise at transonic airspeeds up to Mach numbers of about 0.7. The Boeing 707 and its various derivatives became one of aviation’s most successful commercial aircraft, which saw airline service up until the late 1970s; the aircraft had a capacity of 140 to 219 passengers and a range of 5,750 nautical miles.
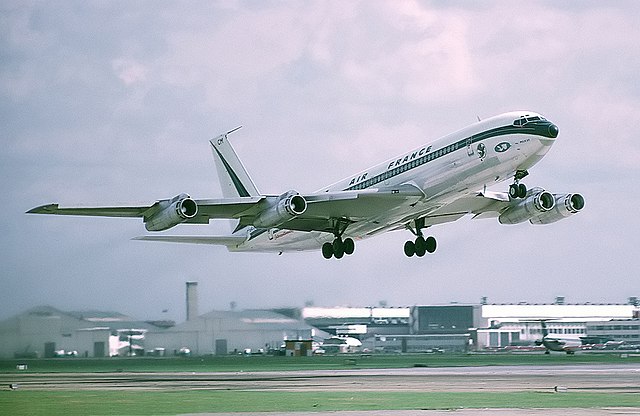
Boeing followed the 707 with a successful series of airliners, including the Boeing 727, 737, 747 “Jumbo-jet,” 757, 767, 777, and the 787 “Dreamliner,” each new model making use of progressively more refined aerodynamic and structural designs, as well as using more powerful and efficient turbofan engines. The introduction of the Boeing 747 in 1969, with its distinctive upper deck shape, was to revolutionize long-haul air travel. The Boeing 747 was to see some lengthening and other shape changes over the years to improve its performance.
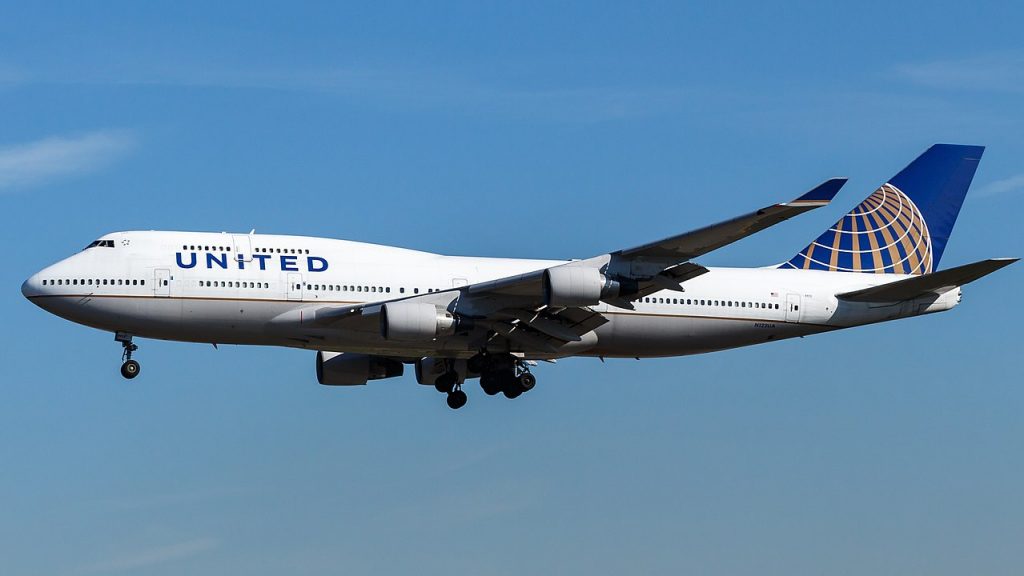
The introduction of the Boeing 787 “Dreamliner” has ushered in a new generation of airliners where a much more significant fraction of the aircraft structure is made of composite materials. Such materials allow for reductions in airframe weight with commensurate improvements in flight range and reductions in operational costs. One advantage of composites is that engineers can tailor the structure’s design to give the needed strength but with a lighter overall construction. The elimination of many fasteners (e.g., rivets and bolts) with composite construction also reduces the empty weight of the aircraft and reduces the likelihood of structural fatigue cracks and the need for repair.
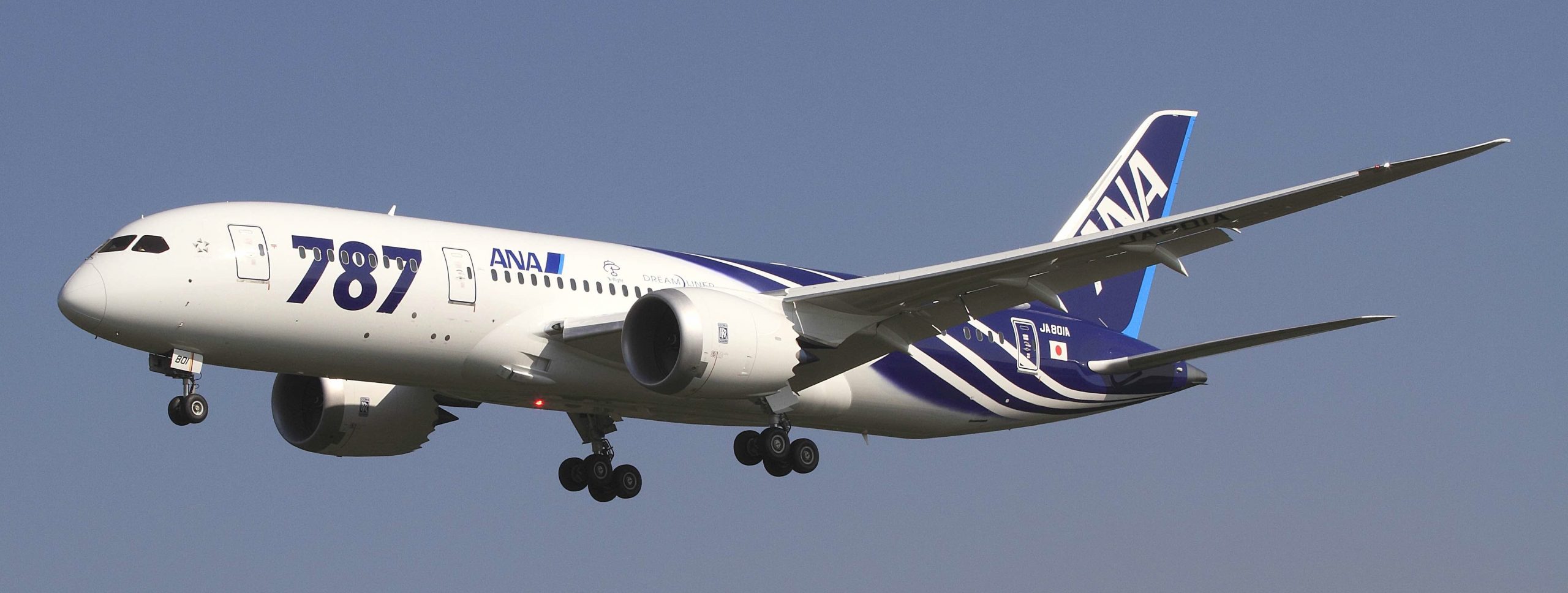
Airbus Industrie has also developed many successful commercial aircraft, including the A300, A320 series, A330, A340, A350, and the giant double-deck A380. One of the latest in the series, the Airbus A350, also uses most composite materials to construct the airframe. It has significantly improved overall performance and reduced seat-mile costs commensurate with the Boeing 787.
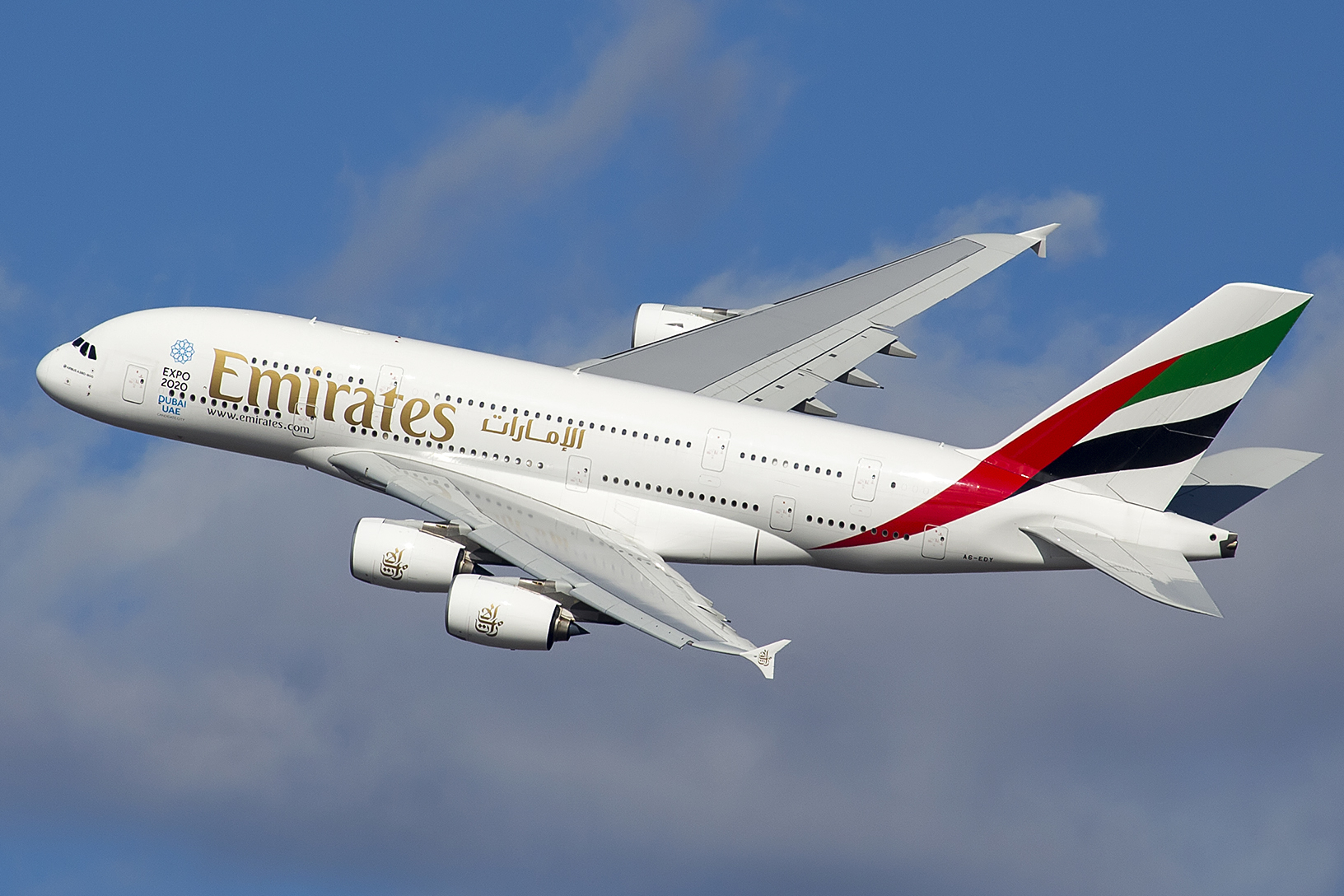
Does aeronautical progress happen in “fits & starts”?
The improvements in complex engineering systems, such as aircraft, do not happen continuously. Instead, performance improvements have historically occurred in irregular bursts or “fits & starts” followed by periods of slow or slower growth or even stagnation, as shown in the figure below.
For example, in the case of the development of the airplane, several technological events can be identified that have led to significant, if not quantum, improvements in flight performance, such as constant speed propellers, the jet engine, swept wings, supercritical airfoils, stronger and lighter materials made of composites, and, more recently, the use of computers and software engineering. However, not all technical developments may lead to immediate improvements; outcomes from fundamental research may take many years of further development and certification for use on commercial aircraft. In some cases, an event that causes a spurt in improvement may be of non-technical origin, e.g., an open competition to reach some performance goal with significant prize money, e.g., the GPB 1,000 prize for the first cross-channel flight awarded to Louis Blériot in 1909, or more recently the $US 100,000 GoFly Prize.
Helicopters & V/STOL
Helicopters are a form of vertical takeoff and landing (VTOL) aircraft. They can take off and land from almost anywhere and hover motionless in the air or fly in nearly every direction at the pilot’s whim, a tremendously valuable capability for both civil and military applications. However, the development of helicopters, a form of rotorcraft, lagged airplanes by almost 30 years, requiring a greater understanding of aeronautical technologies for its ultimate success. Jacob Ellehammer of Denmark was one pioneer of the helicopter, his counter-rotating coaxial rotor machine being photographed in flight as early as 1913, as shown below.
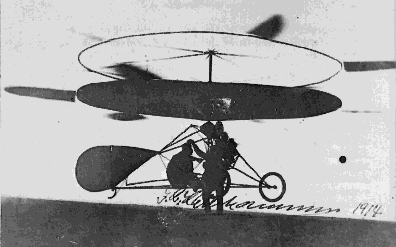
Ellehammer’s machine made brief hops off the ground but could not fly forward or do anything useful. The photographs of his attempts clearly show daylight under all the wheels, confirming the attempt at hovering flight. While Ellehammer did not invent the helicopter, he did make an important and recorded contribution to the advancement of helicopter flight. Paul Cornu and Louis Breguet of France also made notable attempts to build and fly helicopter contraptions between 1906 and 1909. Still, based on the limited photographic evidence, they were all failures. Many other attempts were made to develop and fly helicopters in the following decade, but with no significant success.
The first successful rotating-wing or “rotorcraft” concept was not a helicopter but an autogiro with an unpowered rotor. Juan de la Cierva’s C-4 autogiro flew in 1923, and many other autogiro designs soon followed. The autogiro could take off and land at very low airspeeds, but it could not hover, the aircraft requiring forward motion through the air for the rotor to self-rotate or autorotate. Nevertheless, the autogiro proved to be a testbed for the development of the helicopter, but this was to come about more than a decade later. Indeed, it was Raoul Hafner, about 1936, who integrated Cierva’s flapping hinge rotor design with his cyclic pitch design to make the fully articulated rotor system, which was ultimately the key to the success of the helicopter. However, it was not until the mid-1930s that helicopters became more successful. Laterally, it was not until the end of WW2 that they became a valuable part of the aviation spectrum for military and civil use.
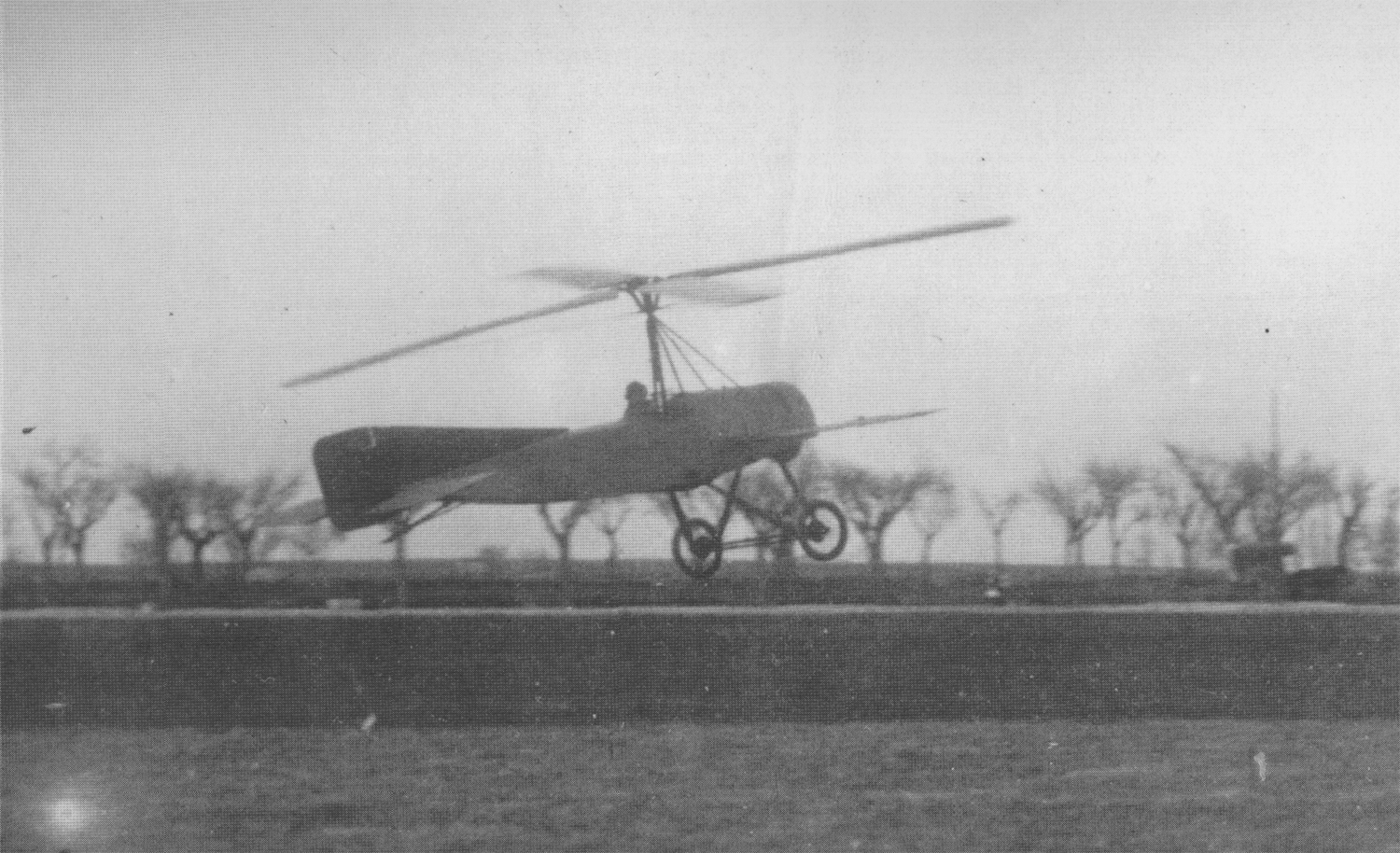
Helicopters saw many advancements in performance and other capabilities during the 1940s and 1950s. The first civil-certified helicopters included the Bell-47, as shown in the photo below, the Bristol Sycamore, and the Sikorsky S-55. The Sikorsky UH-60 Black Hawk, introduced in the late 1970s, would become one of the world’s most successful military helicopters.
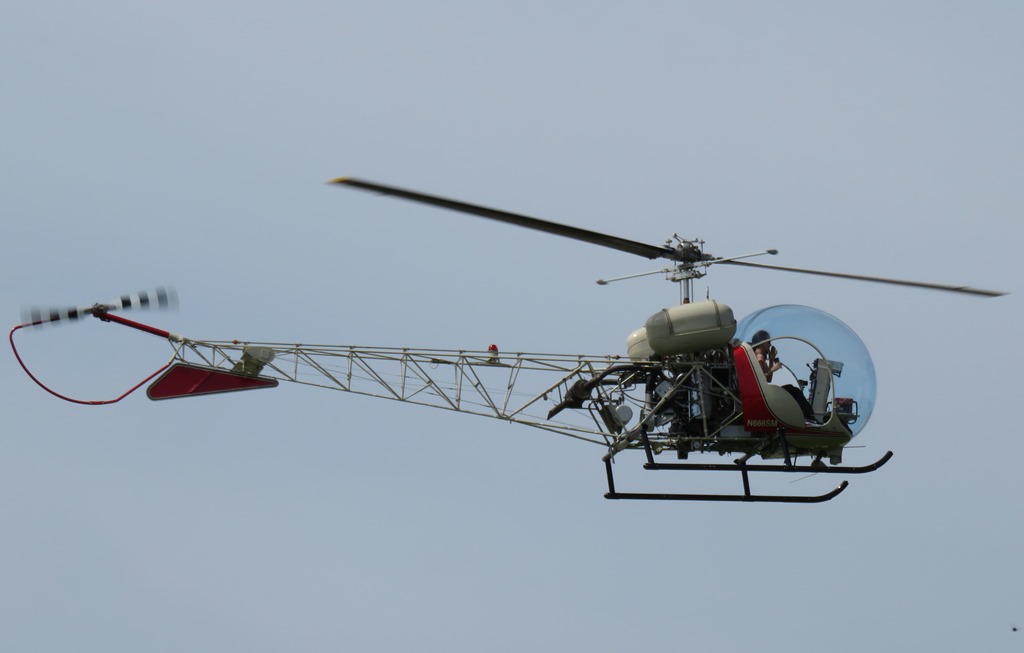
Sustained development in helicopter technology over the last half-century has led to many other successful military and civil helicopter designs. Today, helicopters are increasingly made of advanced composite materials and use various new aerospace technologies to improve their performance, reliability, and safety. The EH-101, as shown in the photograph below, is notable because it uses an active vibration suppression system to minimize the vibratory loads transmitted from the rotor to the fuselage.
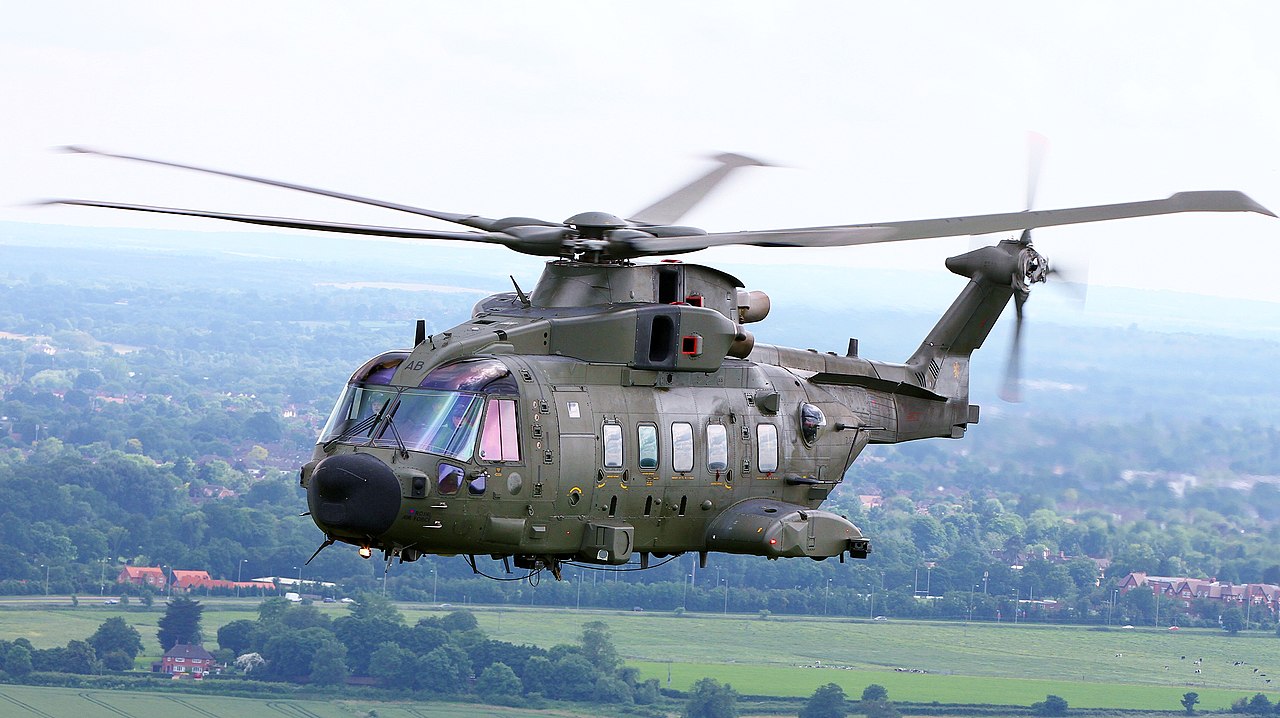
Helicopters are low-speed aircraft with maximum flight speeds of only about 150 knots (173 mph, 278 km/h), which is a limitation caused, in part, by the aerodynamics of the rotor system. In addition, a helicopter is unique in that at higher forward flight airspeeds, the rotor begins to experience both compressibility effects and stall effects at the same time. To overcome such inherent limitations, some helicopters have been fitted with auxiliary fixed wings or a separate propulsion system, which are called compound helicopters. These devices can allow them to fly faster, but usually at the expense of carrying a less useful load. Many uncrewed drones have recently been designed with quad-rotors, allowing vertical takeoff and landing. Although the quad-rotor concept is not new, having been pioneered by Oehmichen and DeBothezat, amongst others, as early as 1907.
Other types of aircraft with vertical and short takeoff and landing (V/STOL) capabilities that can fly faster than helicopters have always intrigued aerospace engineers. Many such V/STOL aircraft concepts have been designed over the decades. As shown below, the so-called V/STOL Wheel of Misfortune catalogs the most prominent attempts to combine the vertical takeoff and landing capabilities possible with a helicopter and an airplane’s high-speed forward flight capability. The Wheel of Misfortune also reminds aeronautical engineers that designing a vertical flight aircraft is challenging and not to “reinvent the wheel” in that “lessons learned” are always a powerful tool to help in engineering design.
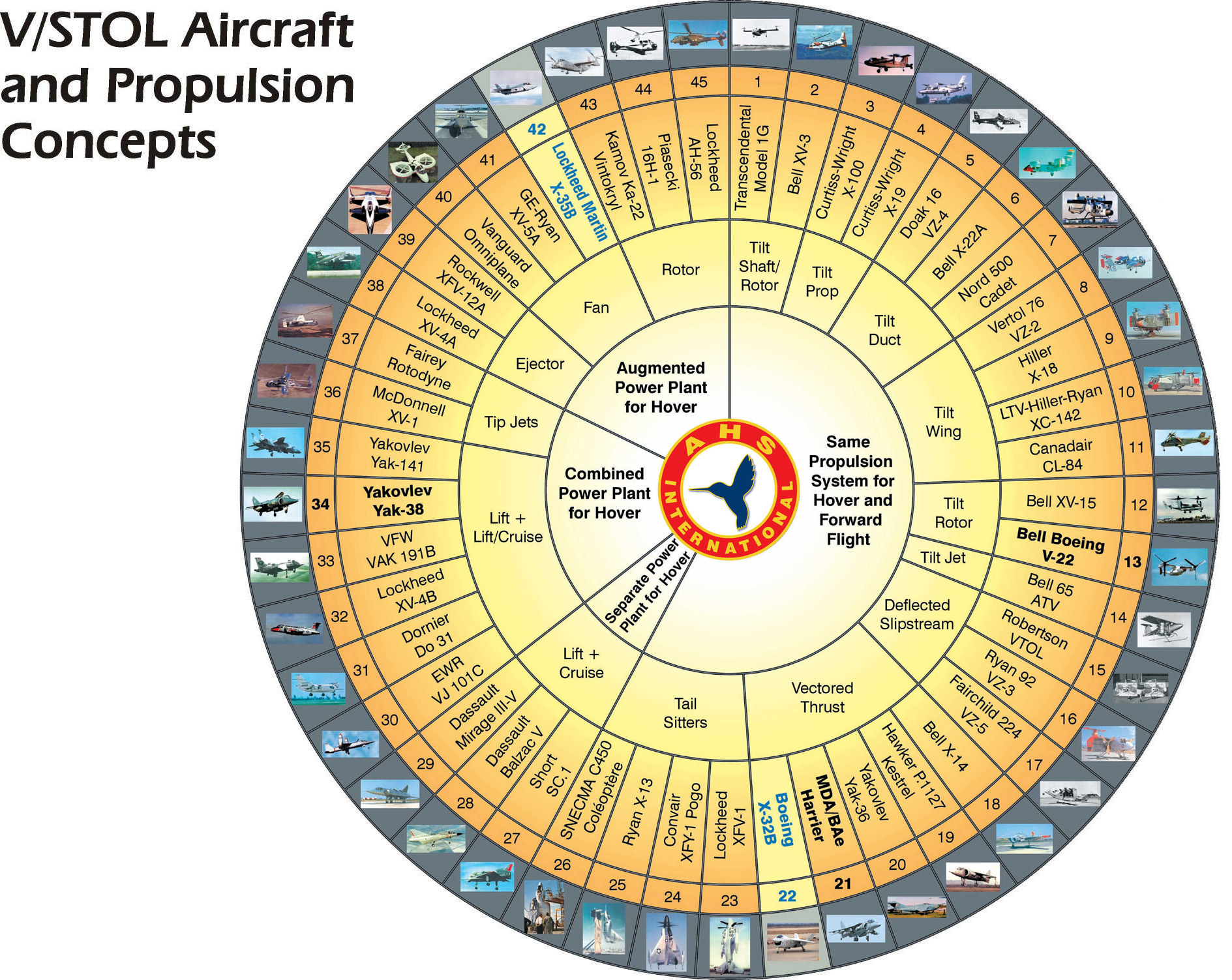
Of these numerous V/STOL aircraft types shown on the wheel that were built and tested, only three have been successful. The best-known V/STOL aircraft is the McDonnell-Douglas AV-8B Harrier, which was developed from an original British Hawker-Siddeley design. In recent years, a version of the F-35 Lightning Joint Strike Fighter has been designed for V/STOL flight, which uses a powerful counter-rotating lift fan in the fuselage. Interestingly, the F-35 (originally the X-35) was the only X-plane to go into production.
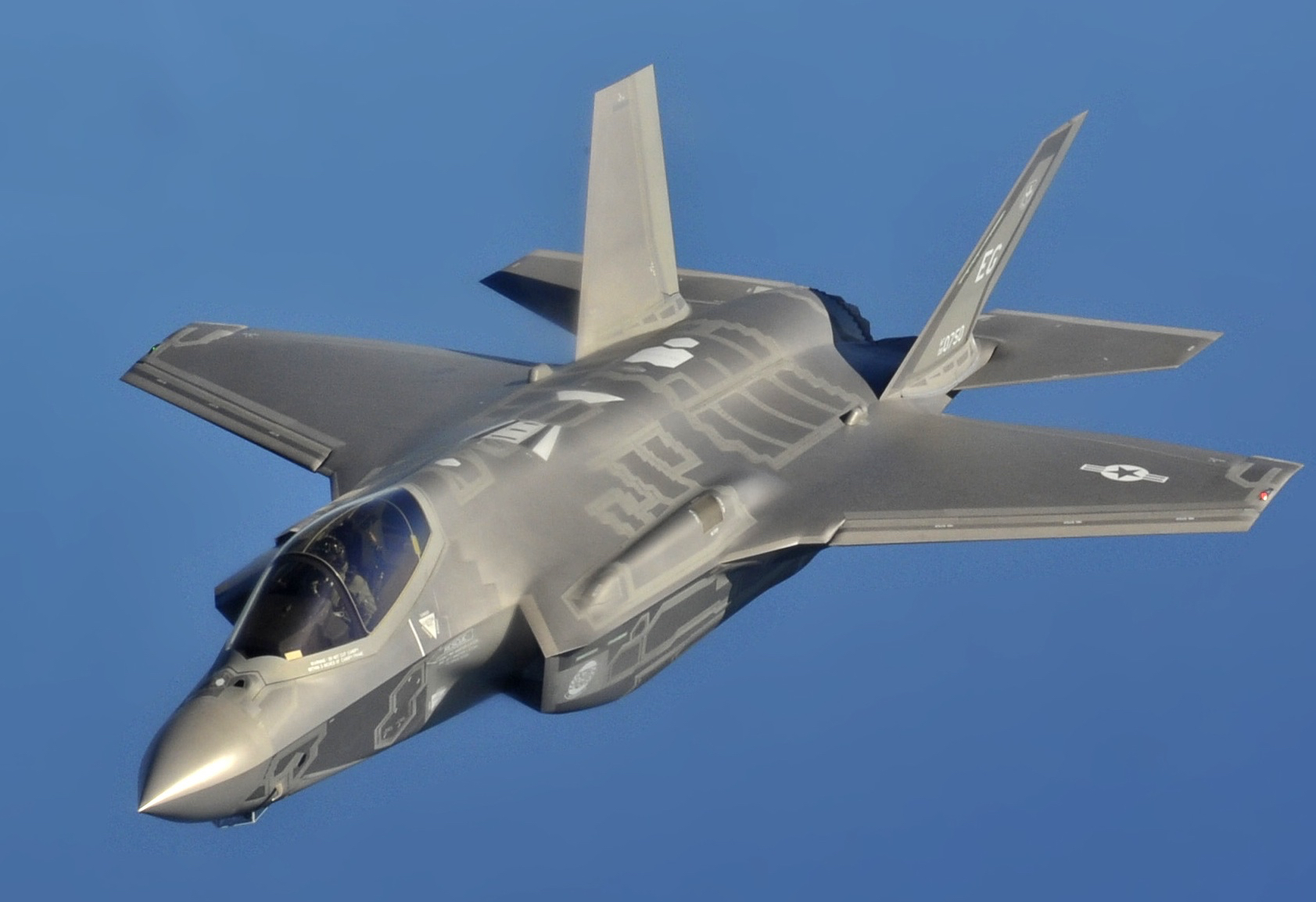
Hybrid rotorcraft such as the notorious Bell-Boeing V-22 Osprey attempt to combine the vertical takeoff and hover capabilities of helicopters with the increased speed and flight efficiency of airplanes. However, such tiltrotor concepts are not as good as a helicopter for the things a helicopter does well (e.g., to hover or operate at low airspeeds) and not as good as an airplane for the things the airplane does well (e.g., flying faster over longer distances when carrying a larger payload). Nevertheless, the tiltrotor concept is integral to the military aviation spectrum. It remains to be seen whether the tiltrotor can ever succeed in the civil marketplace, e.g., in the form of the Leonardo/AgustaWestland AW609, formerly the Bell/Agusta BA609.
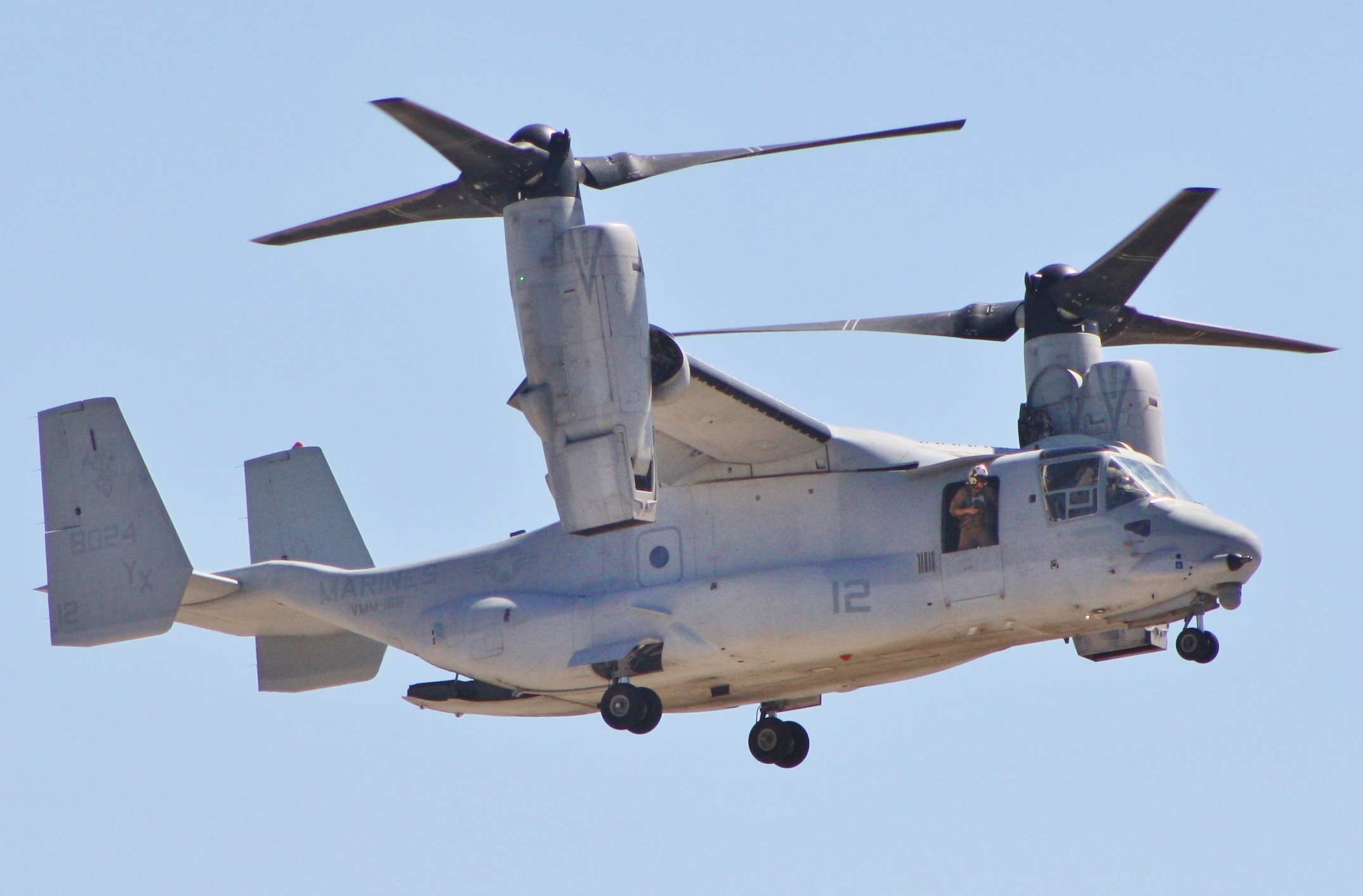
Supersonic Aircraft
The first American supersonic research aircraft was the Bell X-1, a rocket-powered aircraft capable of flying faster than the speed of sound or a Mach number of 1 (approximately 761 mph or 1,225 km/h) in level flight. It was the first of the X-planes, a series of American experimental aircraft designated for in-flight testing of new aeronautical and space technologies. The X-planes were operated by the NACA and later by NASA in conjunction with the U.S. Air Force.
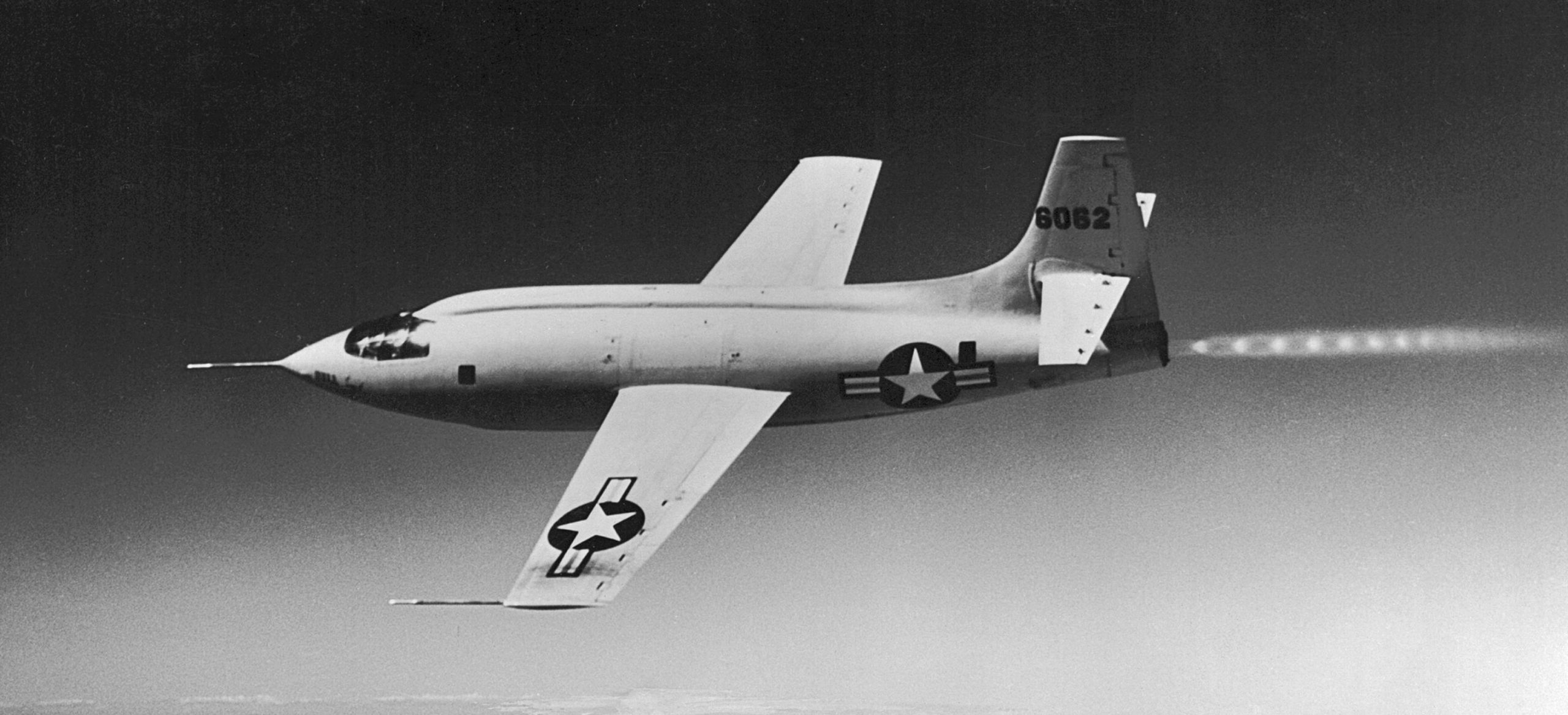
With the introduction of the turbojet engine, new aircraft were designed to fly faster and higher. As a result, it was only a short time before new types of supersonic aircraft were developed. The invention of the afterburner, in which extra fuel is burned in the exhaust of a turbojet engine, gave significant increases in thrust and allowed such aircraft to accelerate through the “sound barrier.” However, the term sound barrier is a misnomer because there is no natural barrier to supersonic flight. It is simply a manifestation of what happened when early airplanes encountered the rapid growth in aerodynamic drag in the transonic flight regime that straddled subsonic and supersonic flight speeds.
A vital feature of these supersonic aircraft designs is thin, highly swept, delta-shaped wing planforms and narrow, streamlined airframes, which allow for efficient supersonic flight. However, a problem is the kinetic aerodynamic heating generated by skin friction at such high airspeeds. This effect raises the airframe’s temperature significantly, causing it to expand, thereby requiring the careful design of the aircraft structure and the selection of the materials from which it is built.
Many types of supersonic aircraft have been developed over the last five decades. Still, nearly all have been used for research and military purposes, primarily as military interceptors and fighter aircraft. One of the most well-known is the McDonnell Douglas F-4 Phantom, which had a top speed of over Mach 2.2. This supersonic airplane could carry many weapons, including air-to-air, air-to-ground, and bombs. Shortly after its introduction in 1959, the F-4 set many world records for speed and altitude.
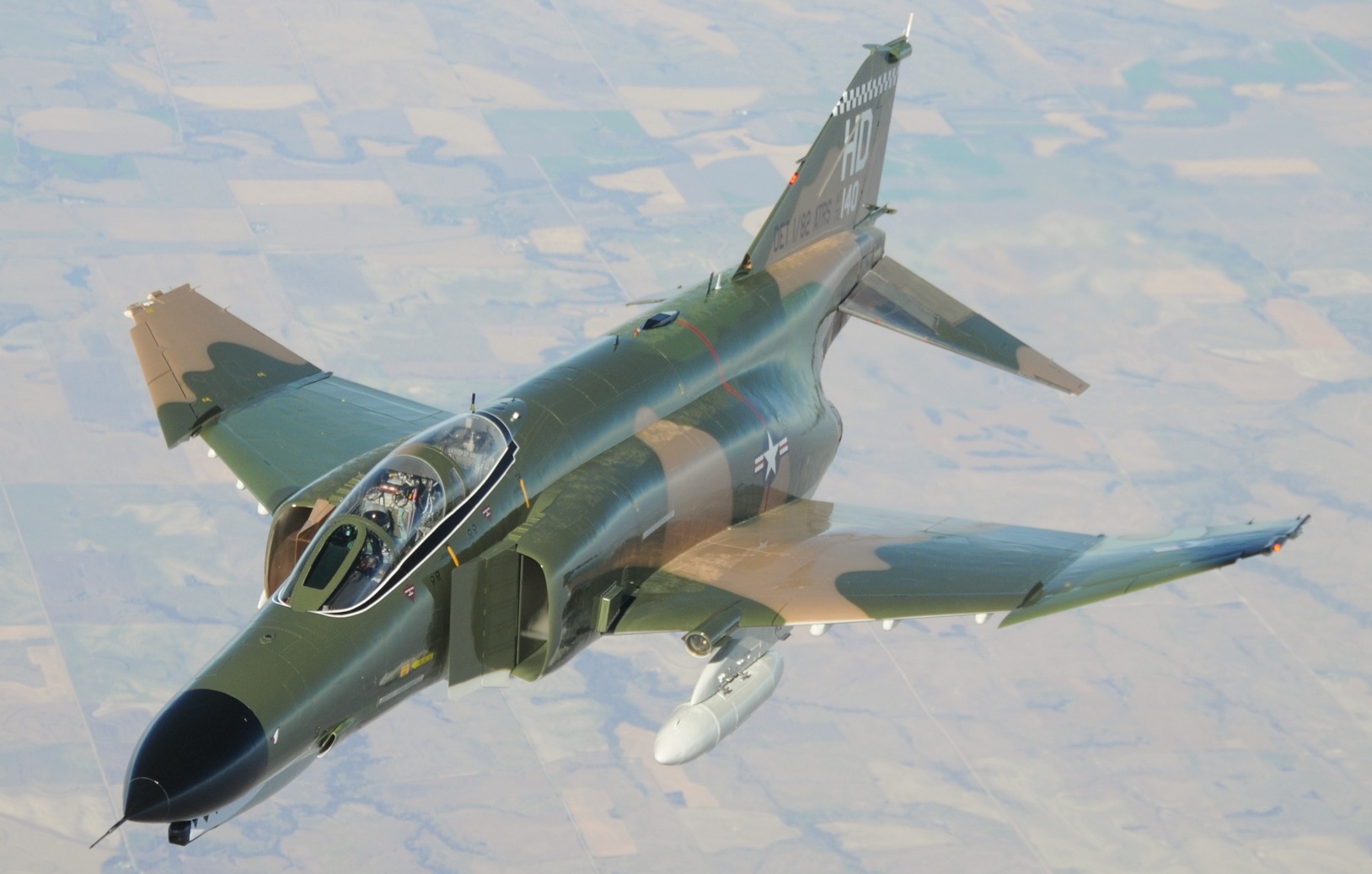
Two civilian supersonic transport (SST) airliners have been built and flown in airline service, i.e., the Anglo-French Concorde and the Russian Tupolev Tu-144. Both were technically successful in achieving sustained supersonic flights with passengers but were economically unsuccessful and have now been retired from operational service. The Concorde cruised at over twice the speed of sound at Mach 2.04 (1,354 mph or 2,180 km/h), with seating for up to 128 passengers.
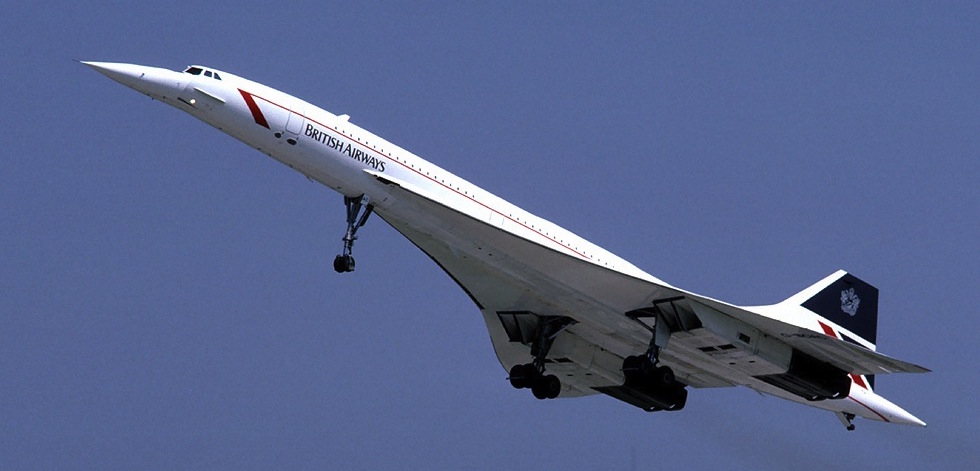
The economics of operating the Concorde was questionable even at the design stage. One reason is that it needed to be bigger to carry enough fare-paying passengers to generate profit for the airlines. The aircraft’s high noise levels during takeoff and landing were also a significant concern when more stringent noise certification requirements were imposed on other (subsonic) aircraft. Boeing also considered developing an SST, the Boeing 2707, but it was not built after it was predicted that even with a larger aircraft carrying more passengers, the economics of an SST for US airline use would be unviable.
Although higher operating costs and low demand from potential passengers have limited the success of supersonic transports, there has been renewed recent interest in building smaller supersonic business jets (SSBJ). While an SSBJ has yet to fly, one or more designs will likely fly by the decade’s end. The appeal of vastly reduced traveling time from a sustained flight at supersonic speeds remains intriguing to engineers and passengers alike.
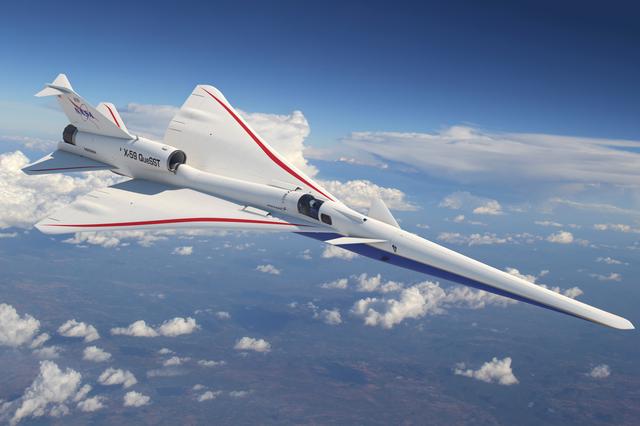
Besides developing the relevant aeronautical technologies to make an SSBJ possible and economically viable, a concern with all supersonic aircraft is the formation of “sonic booms.” Such sonic booms are the sound signature of the aircraft’s shock waves, which project from the aircraft to the ground. The sonic booms produced by a supersonic aircraft sound like two explosions in quick succession; they are intense enough and disruptive to human life that supersonic flight over land is prohibited by civil certification authorities such as the FAA and EASA. The FAA’s regulations can be found in 14 CFR Part 91.817, which prohibits anyone from operating a civil aircraft in supersonic flight over land in the U.S. or from a distance offshore where a sonic boom could reach U.S. shores. Listen here to the sonic booms produced by Concorde as it flew over a ship in the Atlantic Ocean.
However, the problem of sonic booms may not be intractable. With the appropriate design of the shape of the supersonic aircraft, it is possible to lower the sound pressure or “soften” the noise. In 2017, NASA requested proposals to develop its Quiet Supersonic Transport (QueSST) low-boom flight demonstrator. This program called for designing and testing a new X-plane to support future generations of SST aircraft. Lockheed Martin was awarded a contract, the goal being to fly the X-59 QueSST (“Quiet SuperSonic Technology”) in the 2022–24 timeframe. However, it remains to be seen if this goal will be reached.
Regional Aircraft
Large, modern commercial aircraft such as the Boeing 787 and Airbus A350 are sophisticated technological achievements that, in no uncertain terms, are marvels of modern aeronautical engineering and exemplars of the engineering field. In addition, the aircraft industry continues to develop smaller, more fuel-efficient airliners, such as regional jets, which fulfill an essential role in the short-haul marketplace, i.e., for less than 1,000 miles of flight. These “mini-airliners,” such as the Bombardier CRJ and Embraer ERJ series of regional jets, now firmly dominate most short-haul civil aviation markets. They offer the airlines outstanding operational flexibility at a relatively low cost, albeit with some compromises in passenger comfort. They can also operate out of smaller regional airports, providing passengers in many more cities with quick and relatively inexpensive connections to major airports to make longer-distance connections.
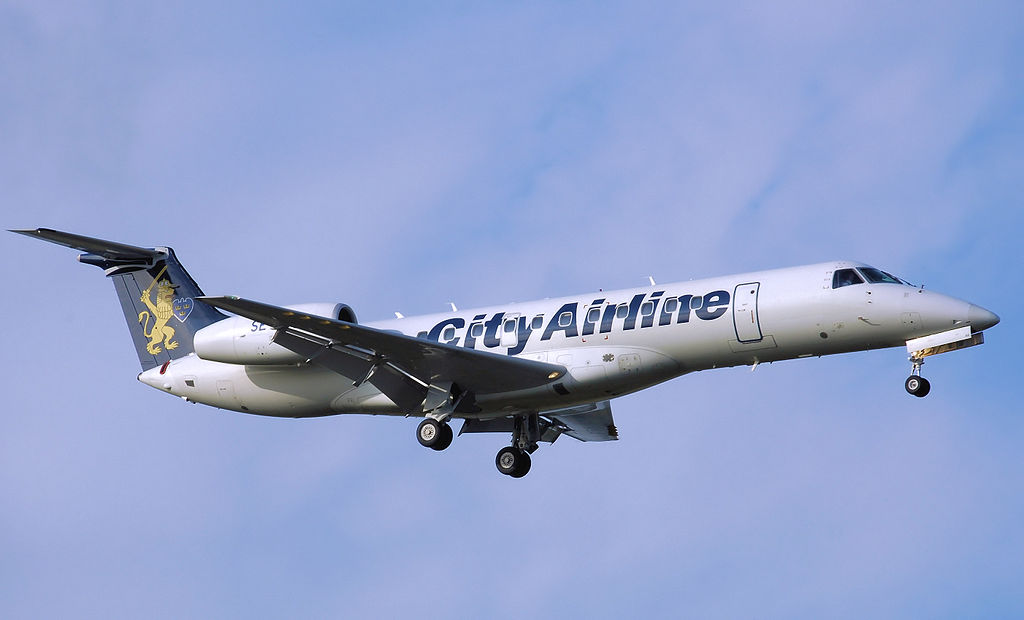
General Aviation
The general aviation (GA) area has seen many advancements since the days of the Piper J-3 Cub. The modern Cirrus SR22 is a low-wing monoplane made of composite materials with a fixed (non-retractable) tricycle landing gear. It is powered by a 310 hp (231 kW) piston engine, and with its smooth and streamlined airframe, this airplane can cruise at over 200 kts (370 kph), even with its fixed landing gear.
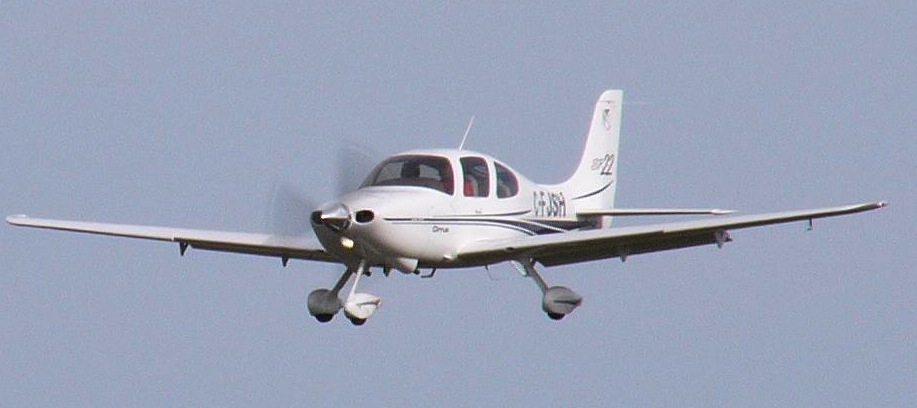
Electric propulsion systems are also seeing rapid developments, particularly for smaller GA airplanes, as shown in the photograph below. Besides the proliferation of electrically-powered Unoccupied Vehicles (UAVs), all-electric aircraft at many scales continue to intrigue aeronautical engineers, and many advancements in this area will likely occur in the future.
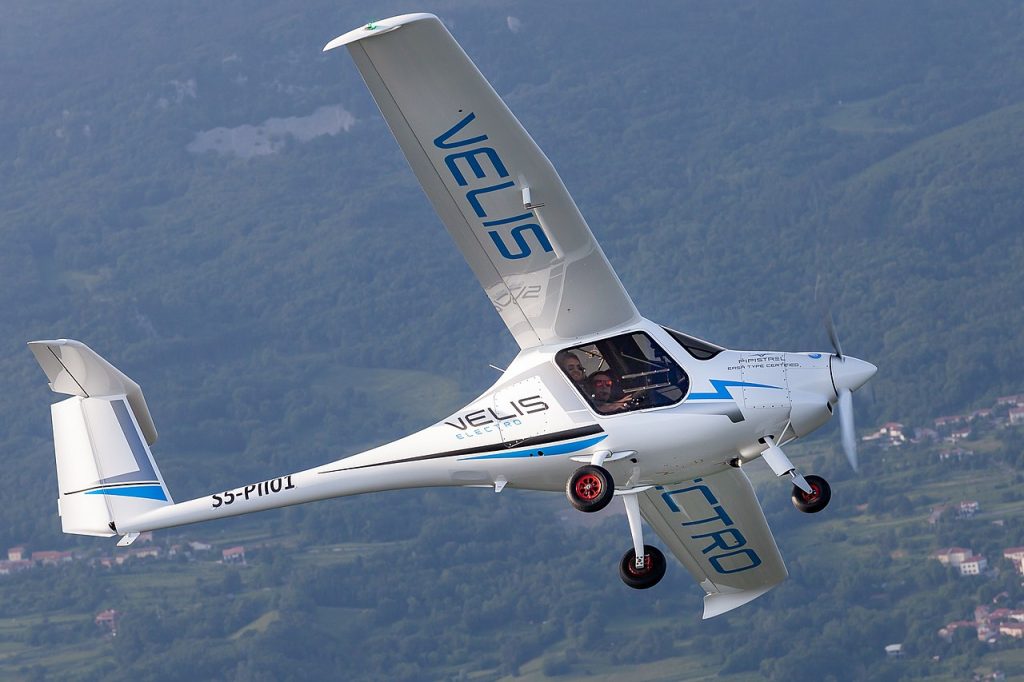
However, the main technical challenges with electric propulsion include the development of battery technology for aeronautical use, mainly to overcome the limitations of their relatively low energy density, i.e., energy per unit weight. Batteries typically have an energy density of about 50 times less than jet fuel, so they are currently ill-suited for larger aircraft. Nevertheless, hybrid-electric power systems will likely provide some advancements in the shorter term. In addition, alternative fuels to jet fuel and gasoline, such as ethanol and synthetic biofuels, are also being explored for use in tomorrow’s aircraft.
Summary & Closure
Over the last half-century, continued research and development by aeronautical engineers have led to many technological improvements in aerodynamics, engines and propulsion systems, lightweight composite materials, flight control systems, software engineering, and alternative fuels. However, further reducing an aircraft’s structural weight and substantially improving aerodynamic efficiency remains critical to reducing fuel consumption and operational costs. The future will likely see further advancements that will take aviation to new safety, reliability, and efficiency levels. It is also likely that electric propulsion systems will see continued developments, particularly for smaller aircraft, driven by improvements in battery technology. The future of aeronautical engineering is very promising, with continued innovation expected to take aviation to new levels of performance, efficiency, and sustainability.
5-Question Self-Assessment Quickquiz
For Further Thought or Discussion
- What is a patent? Why might someone want to apply for a patent? Some people have argued that the process of patenting nearly every development in aviation technology at the beginning of the 20th century, including by the Wright brothers, hindered the advancement of aviation and aeronautical technology worldwide. Discuss this viewpoint.
- While the success of aviation depended on the advancement of engine technology, the success was also because of the development of suitable fuels. Discuss this viewpoint.
- Over the last century, aircraft have been made of wood and fabric, stressed-skin aluminum, and modern composites. Make a list or table highlighting the relative merits of each construction material, including factors such as material costs, tooling, manufacturing processes, fatigue resistance, durability, repairability, corrosion resistance, and crashworthiness.
- Discuss some of the relative advantages of an electric-powered airplane, in general, and for long-distance travel, in particular.
- Review how the development of GPS navigation and collision avoidance systems has improved flight safety.
- Discuss how introducing digital “fly-by-wire” flight control systems on modern aircraft has reduced pilot workload and improved flight safety.
- What is “care-free” handling, and why is this feature is essential for a military fighter aircraft?
- Discuss the considerations of introducing a tiltrotor concept into the civil marketplace. Do you think this is an aircraft that would be used by an airline?
- Research the internet to determine how NASA proposes advancing a new generation of SSTs. What are the main technological challenges? What are the economic and other difficulties?
Additional Resources
There are many online resources to explore aeronautical and aviation history, including information about significant historical aircraft.
- A great movie about the early flight: The Pioneer Era 1900 to 1914.
- Powered Flight and the Wright Brothers.
- Explore the National Air and Space Museum virtually for a close-up look at historical aircraft, and even jump inside some of the aircraft.
- For more aircraft, including the Enola Gay and Space Shuttle Discovery, check out the Steven F. Udvar-Hazy Center virtual tour.
- Browse through the history of aviation on Wikipedia.
- Video on how a rotary engine works.
- An entertaining video on some early aeronautical oddities!
- “History of Flight: Breakthroughs, Disasters and More.” See history.com
- “Aerospace History Timeline” by the AIAA.
- The Jet Story – an early film produced by GE.
- A video on the history of the jet engine.
- “Aviation History” on History.net
- View “Flying Years – History of Aviation” on YouTube.
- A great video on the world’s first jetliner, the De Havilland Comet.
- “Age of the Jet” dates to 1958 and contains historical footage of aviation’s earliest days.
- Video on “X-Planes” on YouTube.
- Documentary from 1990 of the building and test flying of the 727 and 747.
- Sixty years of flight research at NACA/NASA Dryden on YouTube.
- The design of the Supermarine Spitfire – a film from 1944.
- A good book on the V-22 Osprey: “The Dream Machine: The Untold History of the Notorious V-22 Osprey,” available here. It is also available for ERAU users to check out at Hunt Library.